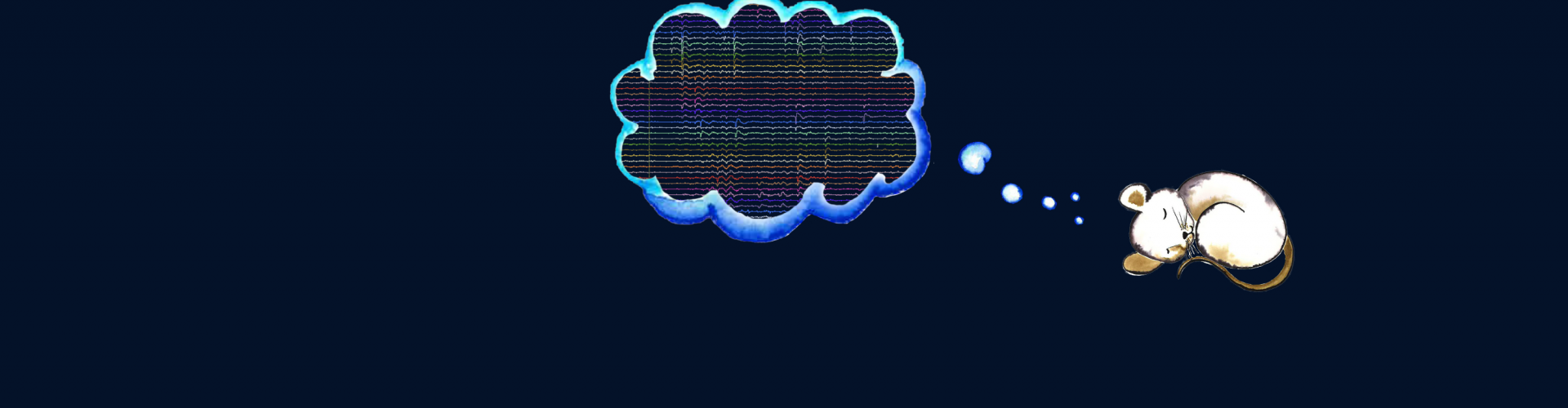
Sleep, Plasticity and Behaviour
Harris Lab
Research Area
Sleep is one of biology’s great mysteries. It is as vital for animal survival as food and water but the reasons for its need are much less clear. Why is it so difficult to understand what sleep is for? Unlike other animal needs, sleep does not involve acquiring anything external to the organism. We think that this provides the first clue about where to look: inside the body, and in particular, the brain.
While we sleep, our brains become active in a variety of ways that are different to the awake state. These specialised neural activity patterns are likely changing neural connections as we sleep. Our lab seeks to understand this daily reorganisation, and to explore what it means to the animal in terms of neural computation, energy efficiency and future awake behaviour.
Research Topics
Each morning that we wake up, our brains are slightly different than the night before. Are they better? Has our internal understanding of the world improved? Are our neurons communicating more efficiently? My lab aims to understand the ways that circuit changes during sleep impact both neural computation and energy use, so that we wake up each morning with brains that are increasingly optimised for our environment.
Our approach is to follow the activity of individual neurons across cycles of wake and sleep. Projects in the lab currently examine how a neuron “decides” which pattern(s) of sleep activity (e.g. slow waves, spindles, sharp wave ripples) to enter into, how participation in a given sleep activity pattern affects that neuron’s connectivity within the network (e.g. synaptic strengthening or weakening), the cellular mechanisms involved in sleep-mediated circuit reorganisation and how these changes influence and refine behaviour across repeated cycles of wake and sleep.
To record neural activity, we use high density silicon probes, in acute and chronic preparations. We employ optogenetic techniques, along with in vivo and in vitro patch clamping to investigate synaptic connectivity, and calculate changes in brain energy use. In vivo vigilance states are measured using implanted ECoG and EMG electrodes, along with LFP recordings.
Our work is largely carried out in the olfactory system, where the well-defined neural circuitry is organised in such a way that it can be hijacked optogenetically to allow for sleep manipulations that would be difficult in other systems. Mice are extremely good at olfactory tasks, as they naturally rely on their sense of smell to detect food sources, locate potential mates, recognise predators and navigate their environment. We can therefore design increasingly complex learning tasks, while maintaining the ability to precisely monitor and control the neurons involved. We use a combination of quick-learning head-fixed tasks with intermittent nap-breaks, and slow-learning freely-moving tasks that require multiple days of experience with intervening periods of sleep.
In addition to investigating how normal sleep impacts the healthy brain, we are interested in understanding what happens when sleep goes wrong. We are currently exploring this in the context of the sleep disruption that accompanies early maternity (in collaboration with Jonny Kohl, Crick Institute) and in the case of disease-related sleeping problems (in Multiple Sclerosis, in collaboration with Nicola Hamilton-Whittaker, King’s Wolfson Institute).