How does the brain encode incoming information to make sense of the world?
Our research in this area focuses on dissecting the neural circuits underlying the processing of sensory information gathered from the sensory organs (the eyes, ears, nose, mouth and skin).
Neuroscientists at SWC also study how the context of a given stimulus, and an animal’s behavioural state, influences perception.
Read more about SWC's Mission
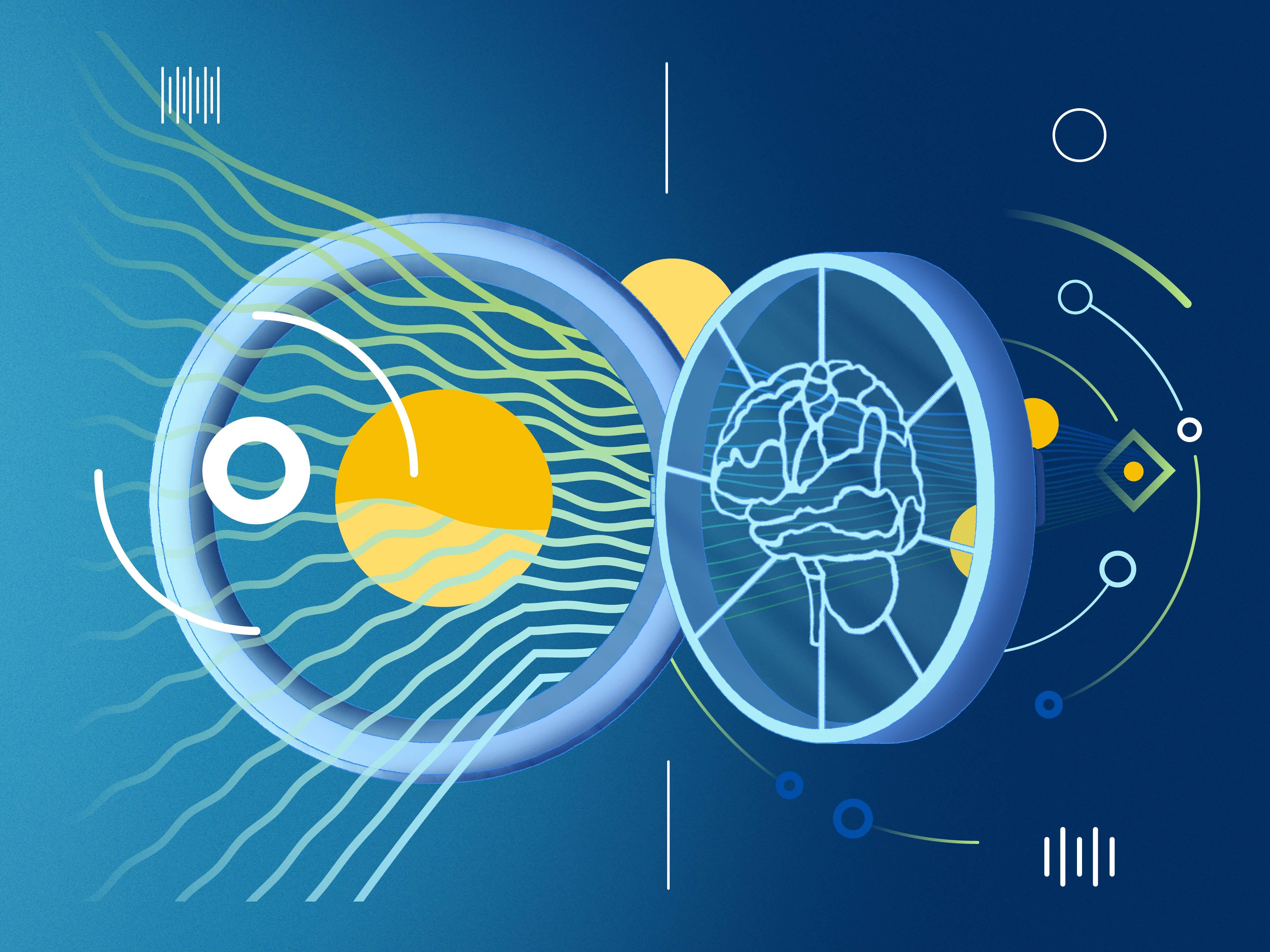
Research News
-
Attention and running influence individual brain cells independently
-
For communication between brain areas, milliseconds matter
-
Unravelling long-range connections in the brain
-
Sainsbury Wellcome Centre researchers reveal mouse and human eye movements share some important similarities
-
Locomotion triggers changes in brain state and long distance communication
-
Neurons that fire together, don’t always wire together
-
Brain-wide tracing of single neurons reveals breadth of information transfer from visual cortex
-
Neuroscientists identify brain circuit that integrates head motion with visual signals