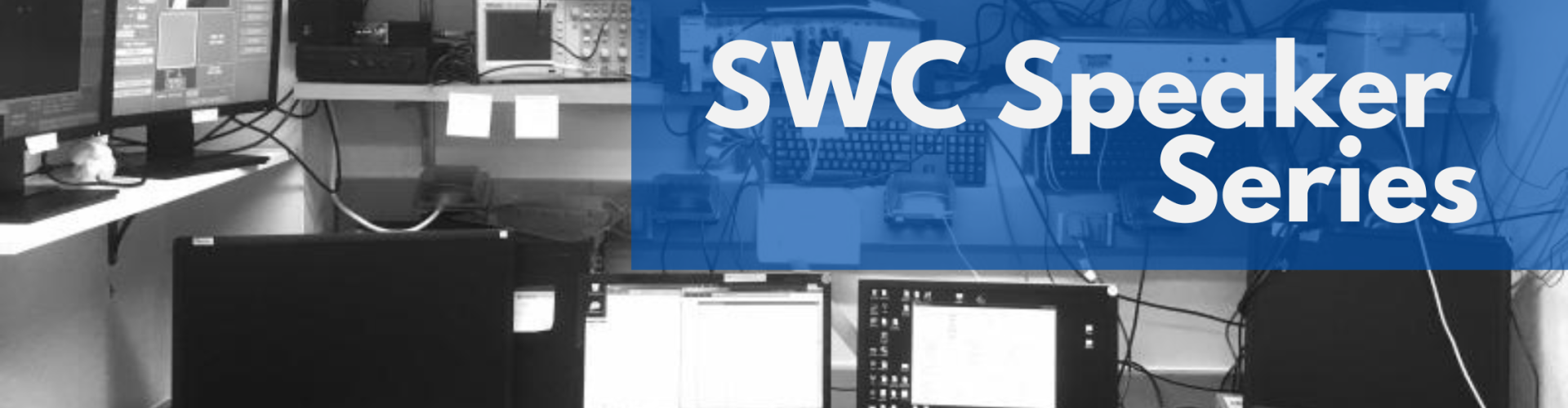
Gaze and cooperative strategy: interareal interactions in the social brain
An interview with Professor Steve Chang, Yale University, conducted by Hyewon Kim
Reciprocal cooperation, like sharing household chores, collaborating on group projects, or pet-sitting, defines the social fabric of life. In this Q&A, SWC Seminar speaker Professor Steve Chang discusses his journey into studying the neural bases of social interaction. He shares his work developing an automated cooperation paradigm for marmosets, discovering different frequency modules for processing rewards in the brain, and more.
How did you first become interested in studying the neural bases of social interaction?
During my PhD work at Washington University in St. Louis (under the guidance of Prof. Larry Snyder), I studied sensory-to-motor transformation, specifically how visual input coming to the retina is transformed into a motor plan to move your arm to touch or reach for a target. We were very interested in reference frames at that time, such as how information is encoded relative to your eyes or hand location. My PhD focused on understanding how neurons in the posterior parietal cortex, particularly the parietal reach region, encoded the upcoming visual reaching target location, determining whether it was eye-centred, hand-centred, or somewhere in between. These studies aimed to find out how information is encoded to guide behaviour.
After that, I thought about other types of reference frames that could be interesting, particularly in social interaction. In social interactions, it's crucial to have a good reference frame for how information is represented. For example, knowing whether information is relative to oneself or another person is essential for smooth communication. Without this understanding, social interaction can become awkward. For instance, knowing that you like coffee and I like ice cream is important for anchoring to self and others, similar to reference frames.
Because of this, I wanted to test whether there is a region or cells in the brain that encode rewards given to another individual. At that time, no study had shown neurons encoding information about another individual purely. So, I moved to Duke for postdoctoral work (under the guidance of Prof. Michael Platt), where we designed an experiment where monkeys made social reward allocation decisions. Sometimes the deciding monkey received the reward, and other times the monkey could donate the reward to another monkey. We identified neurons from various reward-related areas in the brain to see which areas encoded the other monkey's outcome. We considered whether the reward was encoded relative to oneself or someone else.
We found that certain areas were biased toward encoding only one's own reward outcome. However, in the medial prefrontal cortex, neurons clearly signalled the reward outcome of another, even when no reward was received personally. This discovery led me to continue exploring social cognition in my own lab at Yale University.
In what ways are macaques and marmosets prosocial?
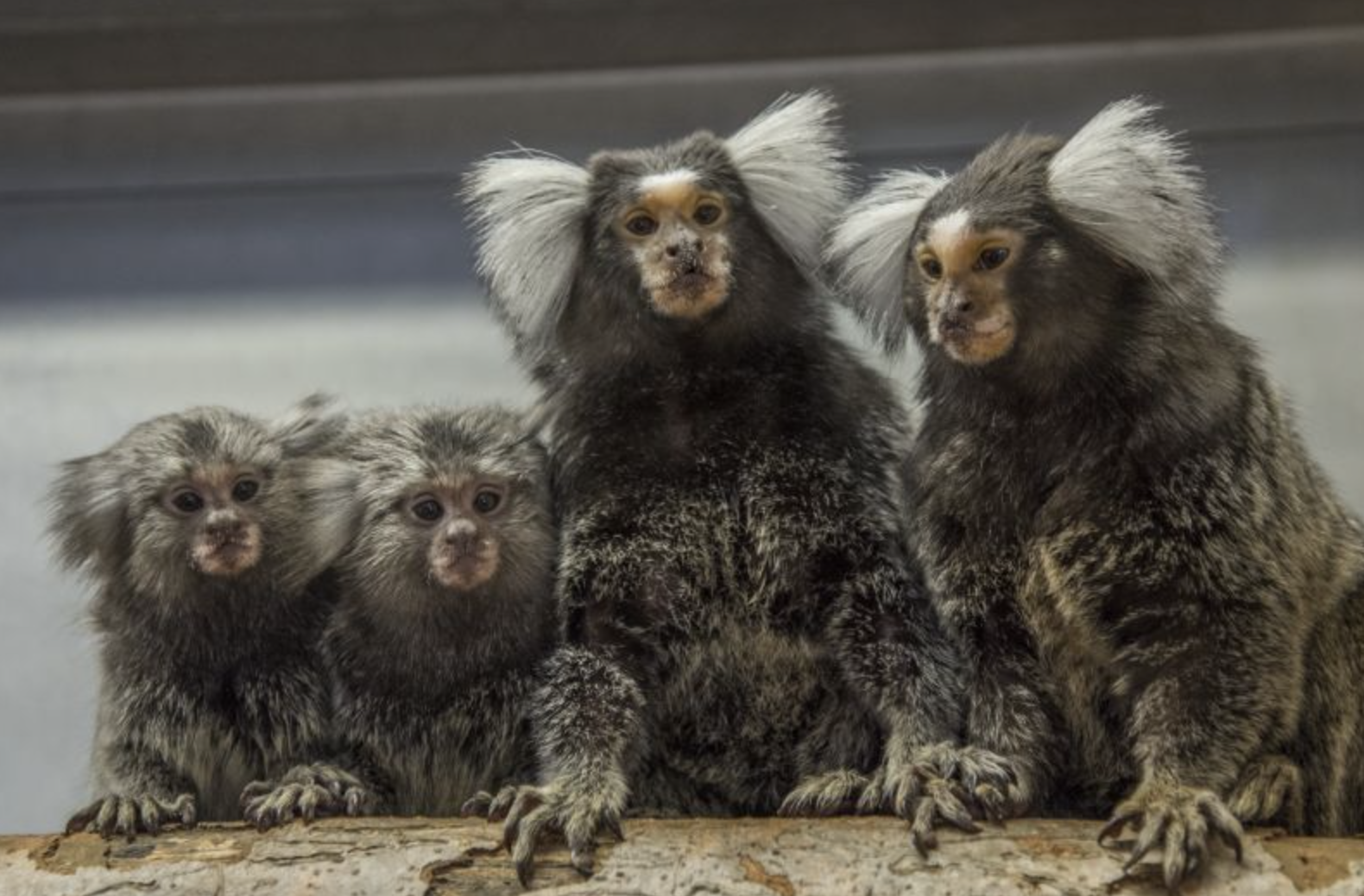
Marmosets are very prosocial. They are cooperative breeders, meaning they take care of the young collectively, not just their own offspring, and raise them together as a group. There is a hypothesis that cooperative breeding has led to cooperation and altruism. Marmosets are also very socially tolerant, making them ideal for studying prosocial behaviour and cooperation.
Macaques, on the other hand, are also very social but in a very competitive way. This is reflected in their ecology; they grow up in large troops, and male monkeys must leave the troop to find a new one and climb the social hierarchy. Resource competition is common, and they are adept at social attention, such as determining if another monkey is looking at them. They engage in prosocial behaviour like grooming, where subordinate monkeys groom dominant ones to gain favour.
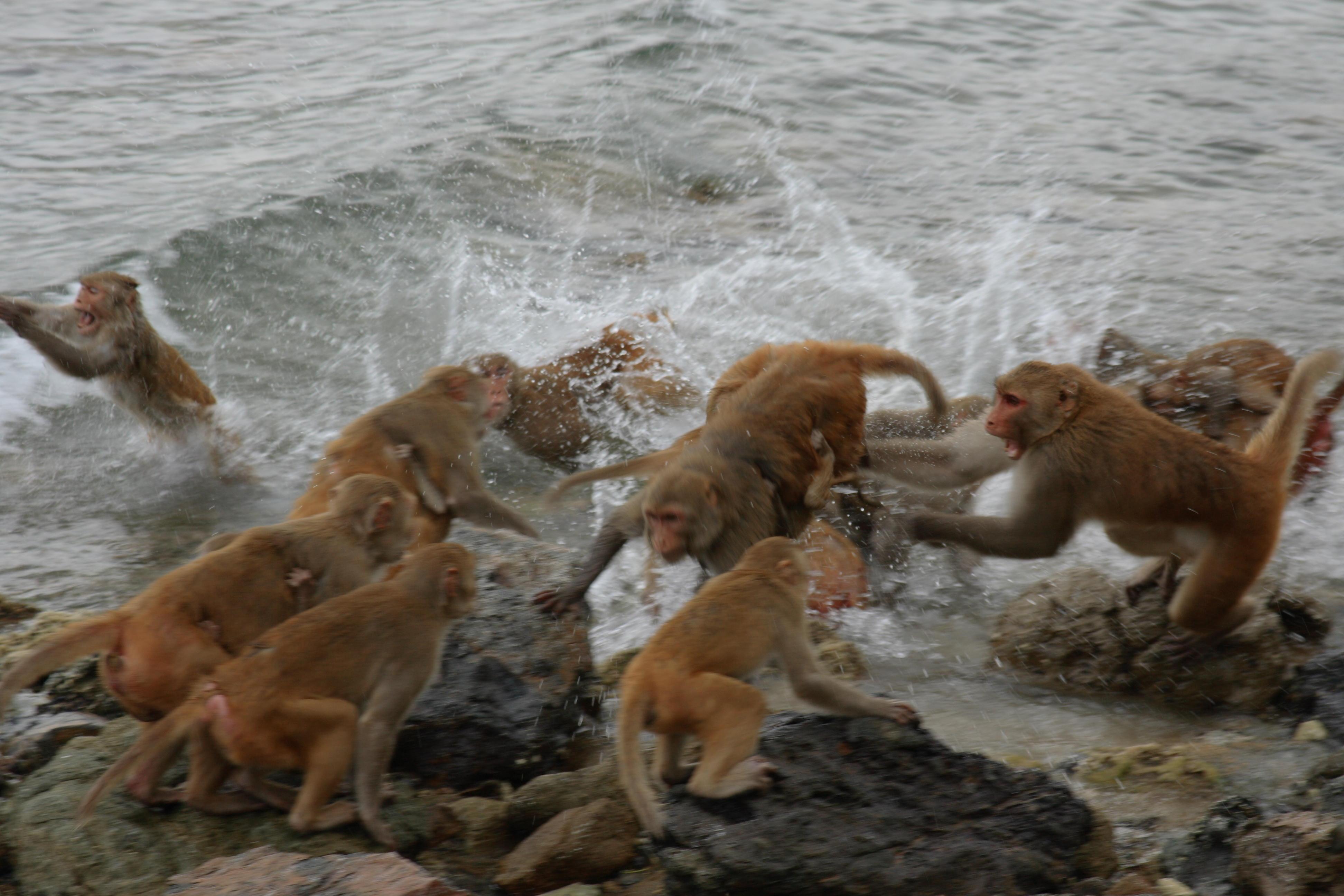
While both species exhibit prosocial behaviour, marmosets display more human-like prosociality, whereas macaques are opportunistic and competitive, with prosocial actions being part of their strategy.
Why did you choose to look at anterior cingulate gyrus and basolateral amygdala in macaques? How about OFC in marmosets?
We focused on the anterior cingulate gyrus (ACCg) because it has the highest number of neurons that encode the outcomes of other monkeys compared to regions like the sulcus of the anterior cingulate cortex (ACCs), which signals the omission of a reward without necessarily indicating if another monkey received it. The orbitofrontal cortex (OFC), on the other hand, mostly signals one's own reward outcome. This led us to consider ACCg as an important region.
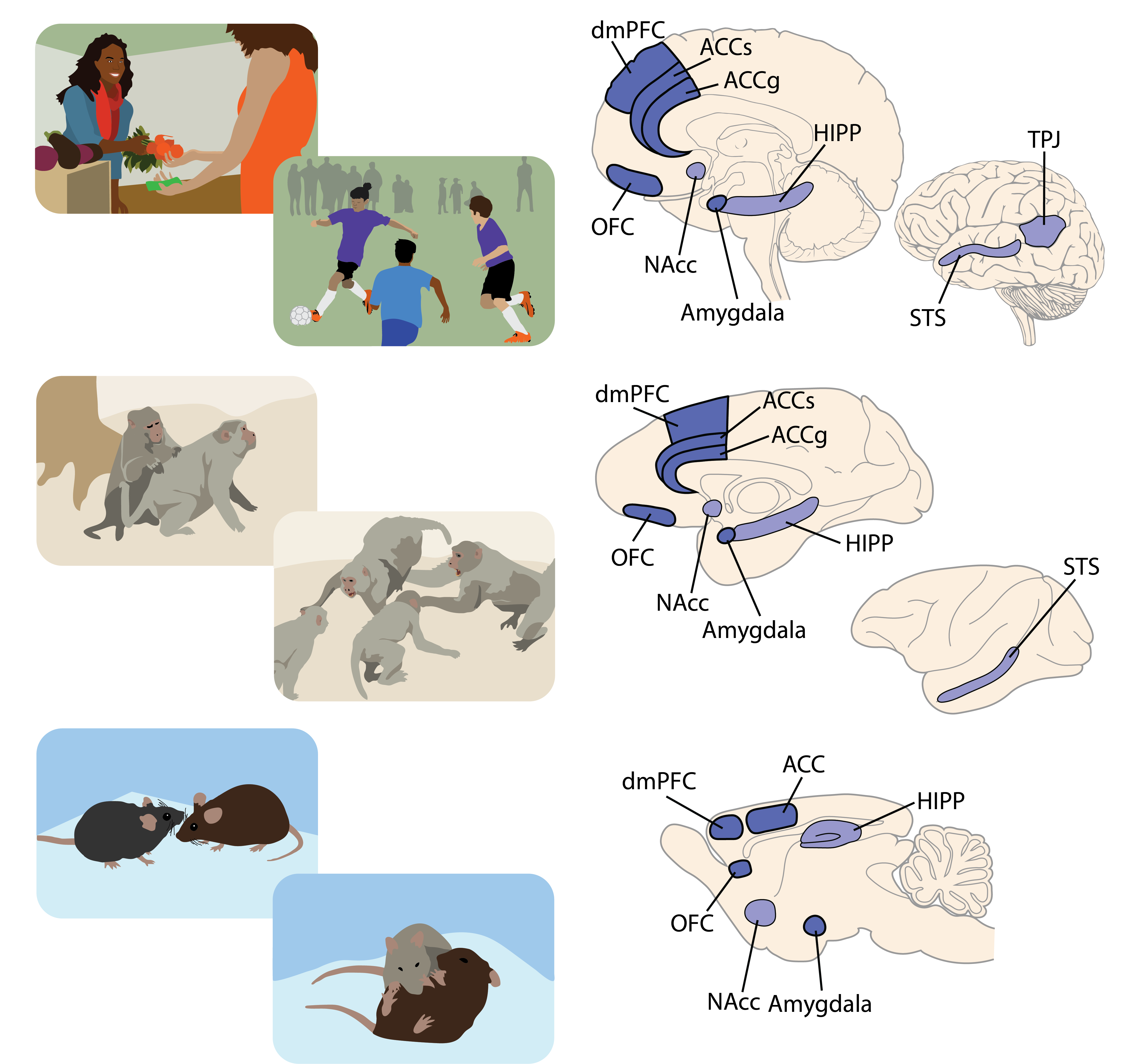
Human fMRI studies have also shown that the ACCg is a specific node in the social brain involved in vicarious learning and reward prediction for another individual, as well as taking advice from others to guide decision-making. This region's activation correlates with empathy scales in humans and negatively with psychopathic scales, reinforcing its significance.
The amygdala was interesting because neurons there encode value for one's own decisions similarly to how they encode value for outcomes affecting another. This suggests a form of value mirroring, which is crucial for understanding its influence on social decision-making.
In human studies, the OFC activation is stronger when earning rewards through cooperation, such as in a prisoner's dilemma task, indicating an extra reward signal from cooperative efforts. This finding prompted us to investigate this area in marmosets during cooperation.
However, studying one brain region at a time can be limiting. Recording from multiple regions simultaneously can reveal how information is shared and routed across the brain. Current research shows that neural coding of behavioural variables is widespread, especially in high-level association areas like the prefrontal cortex. This approach helps to avoid misleading conclusions that can arise from studies focusing on a single region.
What kinds of frequency modules did you find in macaque monkeys making social decision preferences? Could you briefly describe the task?
When we asked monkeys to decide whether to give juice to another monkey or waste it, they preferred to give juice to another monkey. However, when given the choice to either drink the reward alone or with another monkey, they preferred to drink alone, likely for competitive reasons. These contexts highlight a contrast between prosocial and antisocial preferences, even though the monkeys' choices had no impact on their own reward outcome, reflecting true social preferences.
We examined how the ACCg and the amygdala coordinate to guide these preferences. During the expression of these preferences, we observed different frequency channels used for communication between these areas. For prosocial preference, there was increased coherence of amygdala spikes to ACCg local field potential (LFP) in the beta frequency range. This was followed by ACCg spikes coordinating with amygdala LFPs in the gamma frequency range, indicating some separation in the frequency channels used.
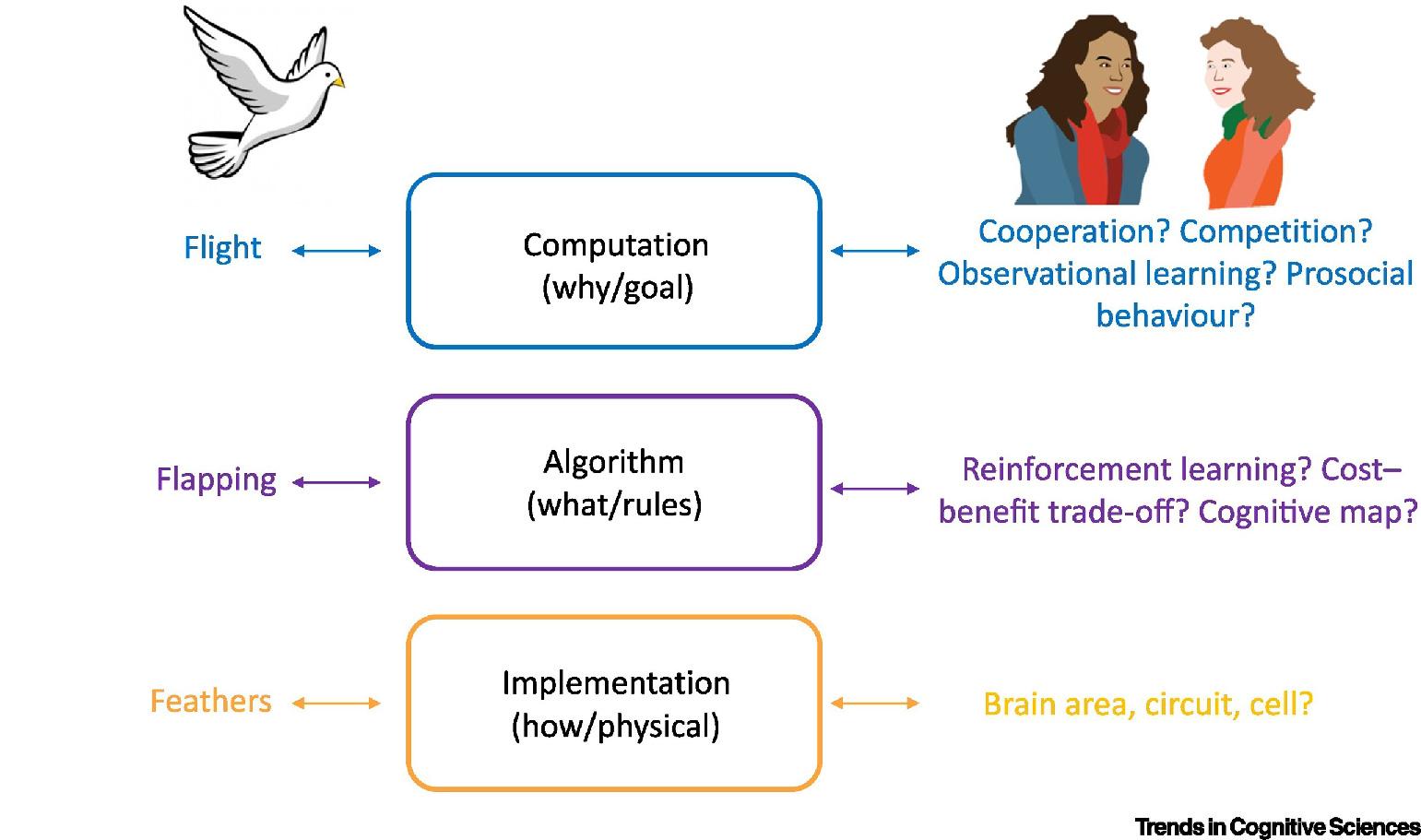
Directionally, information flowed from the amygdala to the ACCg in both cases. When aligning data to the time of reward, we compared how the two areas coordinated when processing self-reward versus vicarious reward. Interestingly, the same ACCg spikes and amygdala LFP sites were involved, but the synchronization frequency differed. For self-reward, synchronization occurred in the alpha-beta frequency range, whereas for vicarious reward, it shifted to the gamma frequency range. This suggests that different frequency modules are used to prevent interference between the two types of information, akin to tuning a radio to separate stations.
Were you surprised to find this division in frequency modules and this so-called radio-like transmission system?
I was surprised because I initially thought that the level of information coordination might be higher in one condition than the other. However, the discovery that different frequency ranges are used for different types of information was unexpected. It makes sense though, since using different frequency ranges prevents interference. This might reflect how information is routed in the brain, avoiding conflicts or noise by not transmitting the same information through the same channels. This kind of frequency modulation might be indicative of how the brain directs information to different areas.
How did you create a naturalistic cooperation paradigm for neural investigation of social interactions in marmosets?
Primatologists have long used marmosets to study cooperation through intuitive tasks like rope pulling. In these tasks, it's too much for one monkey to pull the rope alone to get the reward, so they must work together, each pulling the rope to receive the reward. However, this setup has limitations, such as the monkeys only performing the task 10 to 15 times a day and requiring human intervention to reload the tray each time, making it time-consuming and challenging for neuroscientific studies.
To address this, we developed an automated paradigm called the Marmoset Apparatus for Automated Pulling or ‘MarmoAAP’. This system allows marmosets to work together to pull a lever and receive a liquid juice reward. They can perform around 150 trials in a given session, providing ample data for brain studies. The setup is naturalistic, as the marmosets are not constrained to perform the task if they don't want to, allowing for spontaneous coordination and a rich array of behaviours before and after the cooperative pulling.
Modern advances in computer vision enable us to track their social gaze in 3D without markers, linking social gaze dynamics to cooperative pulling behaviours. Remarkably, marmosets learn this task quickly without much training, allowing us to test various social relationship pairs. This setup lets us explore the effects of dominance, sex, and familiarity, offering a rich dataset to study how the brain facilitates cooperative interactions in a naturalistic environment.
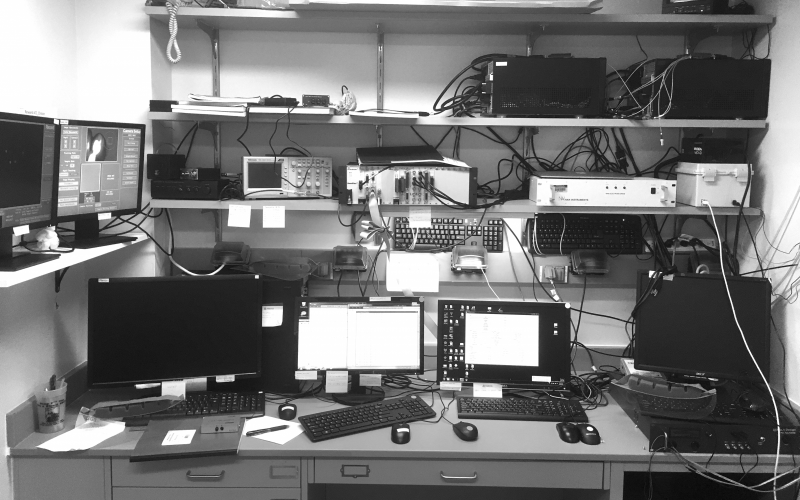
Sometimes, when we as humans observe animal behaviour and it seems like they are cooperating, it can be a significant hurdle to convince ourselves that they are genuinely working together. Even when it appears obvious, establishing that they are truly cooperating requires a lot of work. We are excited about this paradigm as all the evidence points to them working together for mutual benefits.
Can you share more about markerless behavioural tracking in freely moving marmosets?
This work builds on prior inventions like DeepLabCut and Anipose, which allow tracking in three-dimensional space. By training a model to recognize different markers in video frames, once trained, it can track these markers in new videos. This capability enables us to track social gaze directions and movements, thanks to advancements in computer vision.
How do these animals use social gaze strategically? Were you surprised by these findings?
There are several instances where social gaze is crucial in cooperative interactions. One instance is when two animals are pulling but are too far apart and don't get rewarded, as they need to pull within one second. They use gaze to communicate and synchronize their pulls. Another instance is when the dominant monkey pulls and looks at the other monkey to ensure continued engagement. Additionally, before engaging in pulling, they use gaze to communicate and coordinate their behaviours, indicating when to start working together. Social gaze thus becomes an essential tool for sending and receiving information in these cooperative tasks. It seems to serve a social monitoring role here.
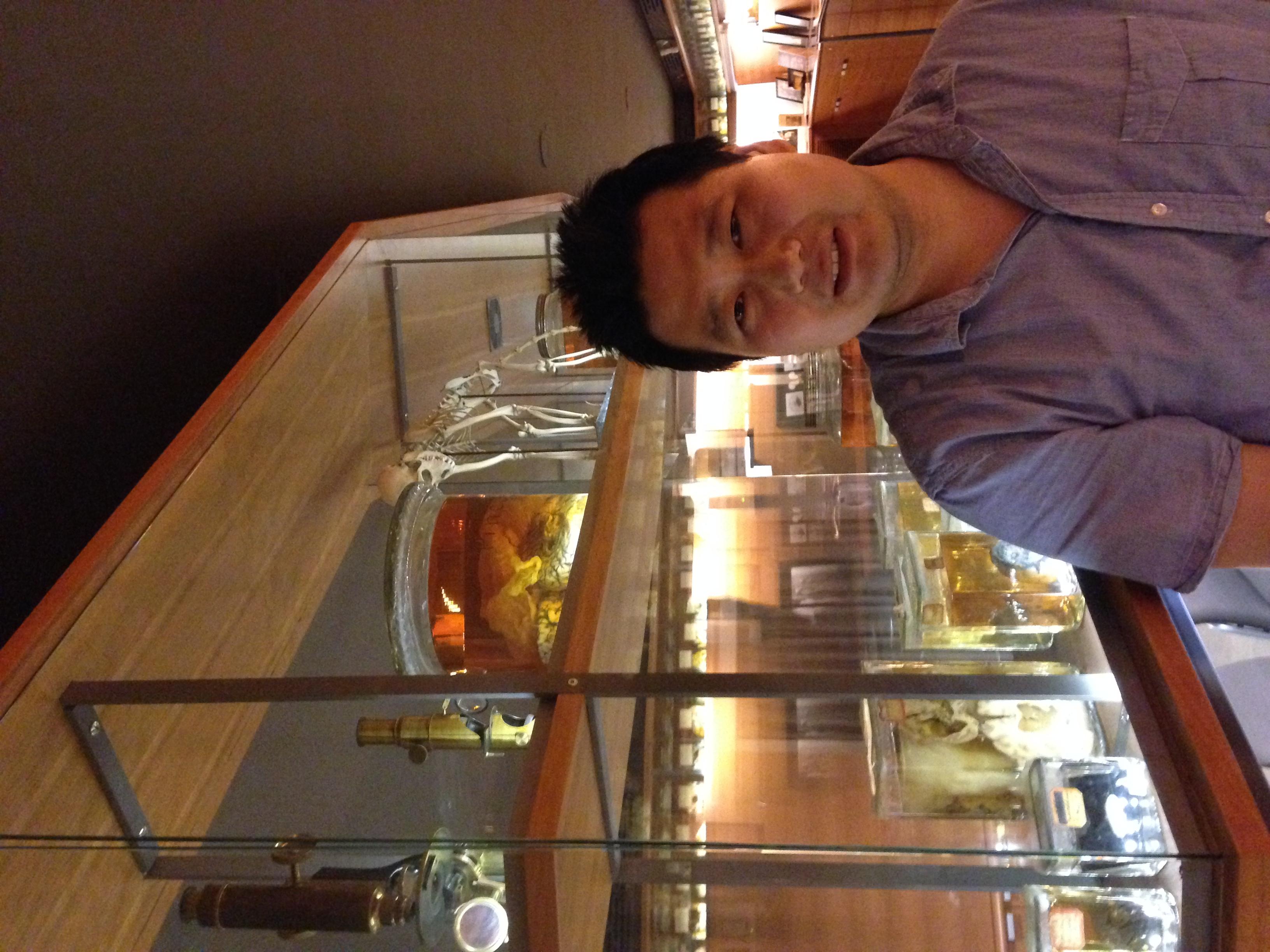
In the wild, marmosets use head gaze extensively to communicate. They also rely heavily on vocalizations, but head gaze is a typical attentional cue for them. It is commonly used for both sending and receiving information, similar to how humans use eye contact for non-verbal communication. This head gaze behaviour serves as an important method for marmosets to interact and coordinate with one another.
What is your current hypothesis for interareal interactions in the social brain?
The social brain encompasses many regions, each sharing representations of social behavioural variables, yet also having some specializations. Brain regions in the temporal cortex and more posterior areas are more involved in the perceptual side of social behaviours, whereas social brain regions in the prefrontal cortex are more involved in decision-making. These regions must exchange information, and this inter-area coordination allows for routing information from different nodes of the social brain, enabling them to function as a larger network. The coordination patterns of activity likely reflect how this information is routed and communicated within the brain.
Could your work have implications for humans, clinically or generally?
One of the most relevant applications of our research is in understanding autism. Cooperation, especially reciprocal cooperation, is impaired in autism, leading to difficulties in reciprocating cooperative behaviours after receiving cooperative gestures. This deficit in reciprocity is linked to certain brain regions we study, such as the medial prefrontal cortex and amygdala, which are also implicated in autism.
Studying the basic mechanisms of social interaction can help us understand how these interactions differ in conditions like autism. Marmosets offer promise for this research, as we can subject them to cooperative paradigms and observe the effects, particularly in relation to genes like Shank3, which are implicated in autism.
In macaque studies, social attention is a clear area affected in autism, with individuals having trouble maintaining eye contact and engaging in social gaze interactions. Understanding the neural networks involved in social gaze and pro-social preference can provide valuable insights into the disruptions seen in autism.
In fact, our lab has a branch dedicated to studying social gaze interactions between macaque monkeys, observing their natural interactions and how they use gaze to communicate with each other. These studies contribute to our understanding of social cognition and behaviour in both humans and non-human primates.
What are some next steps you’re excited about?
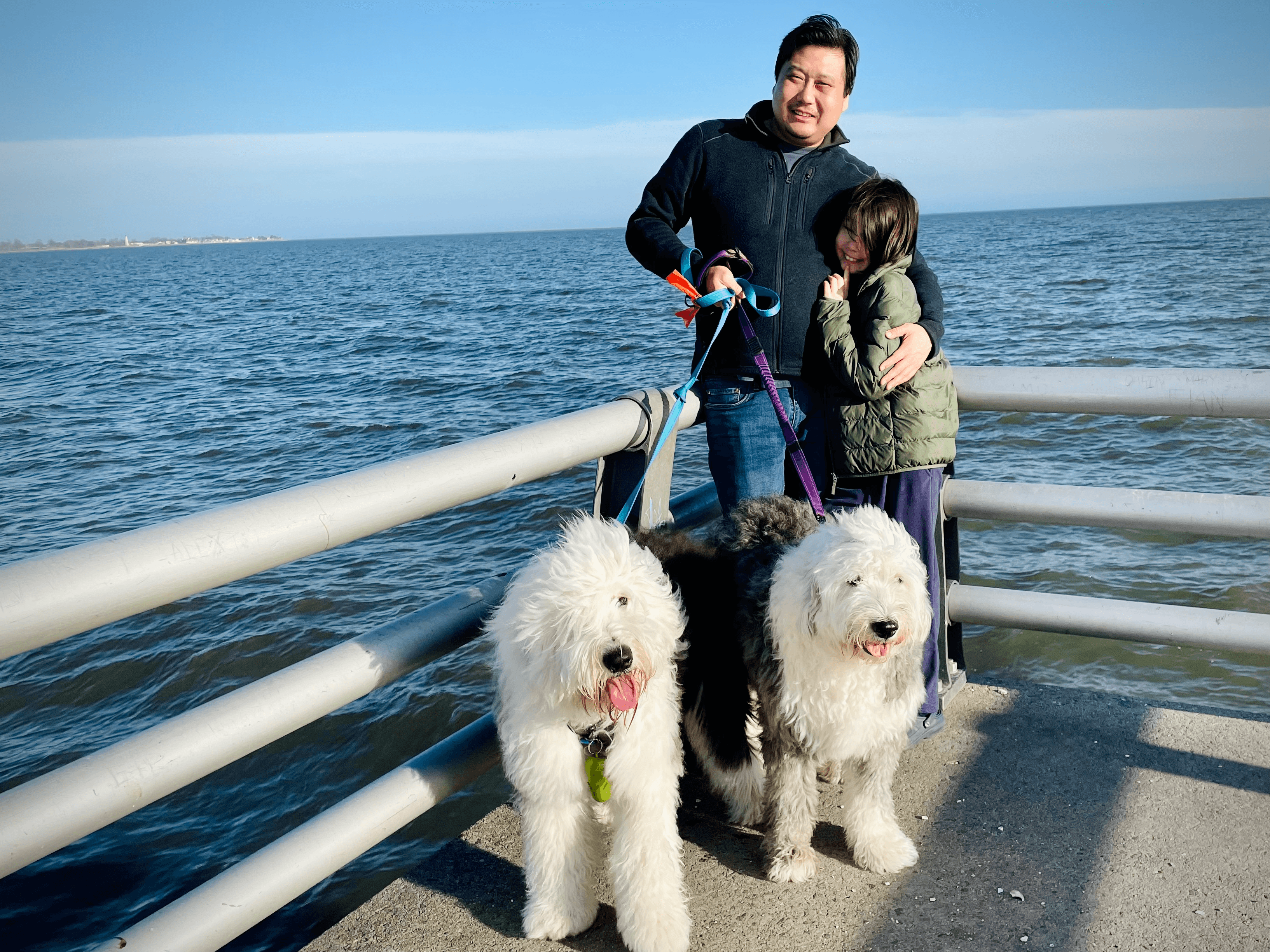
In our research with marmosets, we aim to explore different types of cooperation beyond mutual cooperation. One area of interest is reciprocal cooperation, where individuals switch roles after a few trials, working together for mutual benefit. Additionally, we investigate social motivation by varying the effort levels required for cooperation, aiming to understand the behavioural and neural mechanisms underlying prosocial motivation.
On the macaque side, we focus on internal states that influence social behaviour. While monkeys have personal and antisocial preferences, these preferences are not static and can vary within a day. We seek to understand the fluctuations in social preferences and what factors guide these variations. By studying the natural fluctuation of social states, we hope to gain insights that could inform interventions and predict when individuals may be more or less prosocial.
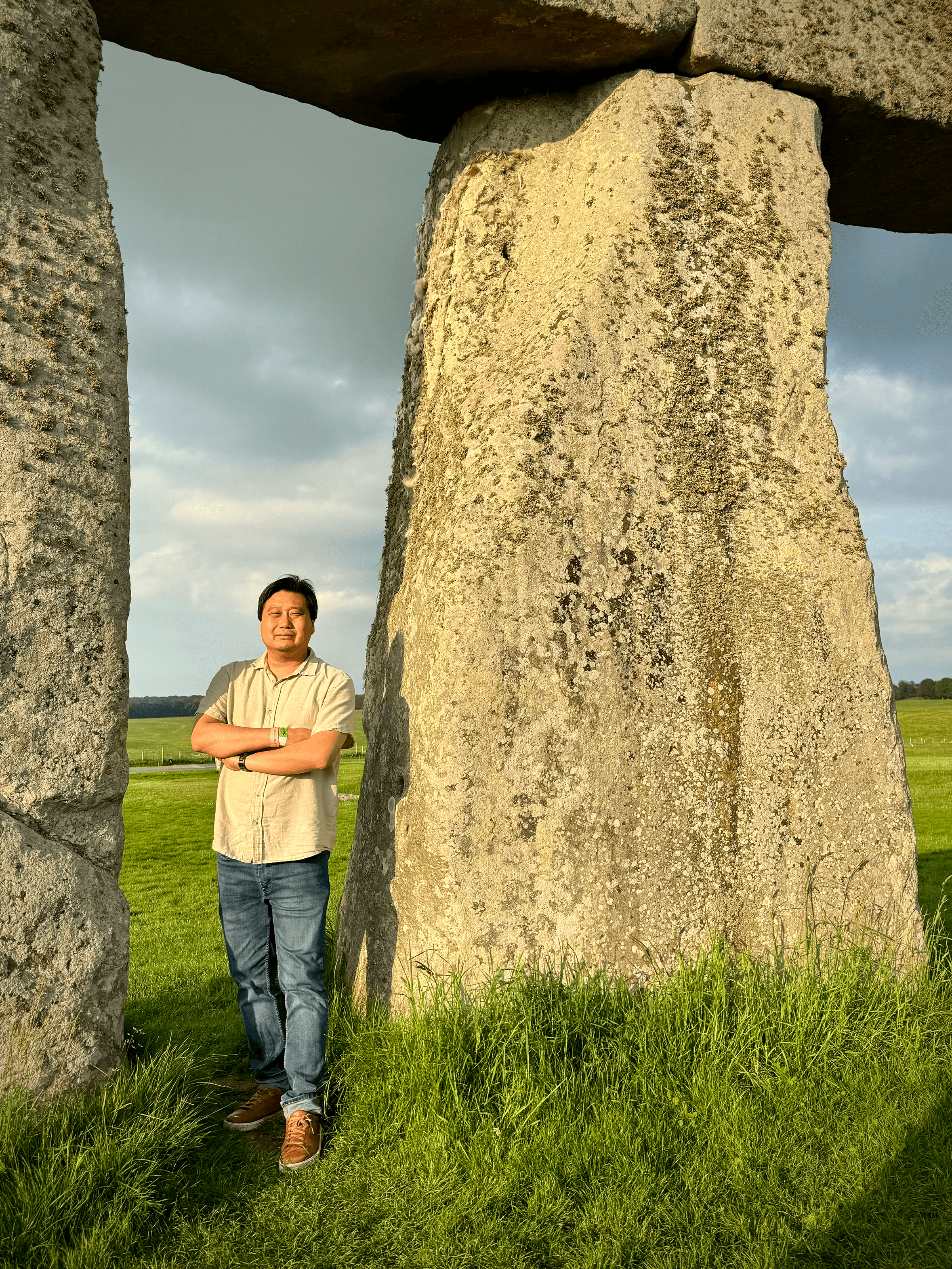
About Professor Chang
Steve Chang is an Associate Professor of Psychology and of Neuroscience at Yale University. He is also a member of the Wu Tsai Institute and the Kavli Institute for Neuroscience at Yale. He is the co-Director of Undergraduate Studies of Yale's Neuroscience (NSCI) major. His research aims to understand the neural circuit mechanisms of social cognition and social decision-making. Major research approaches include using naturalistic social interaction paradigms combined with state-of-the-art behavioral and neural technologies. The ultimate goal of the research program is to elucidate the neural mechanisms underlying social cognition and to learn how these processes may be disrupted in psychiatric conditions with social deficits.