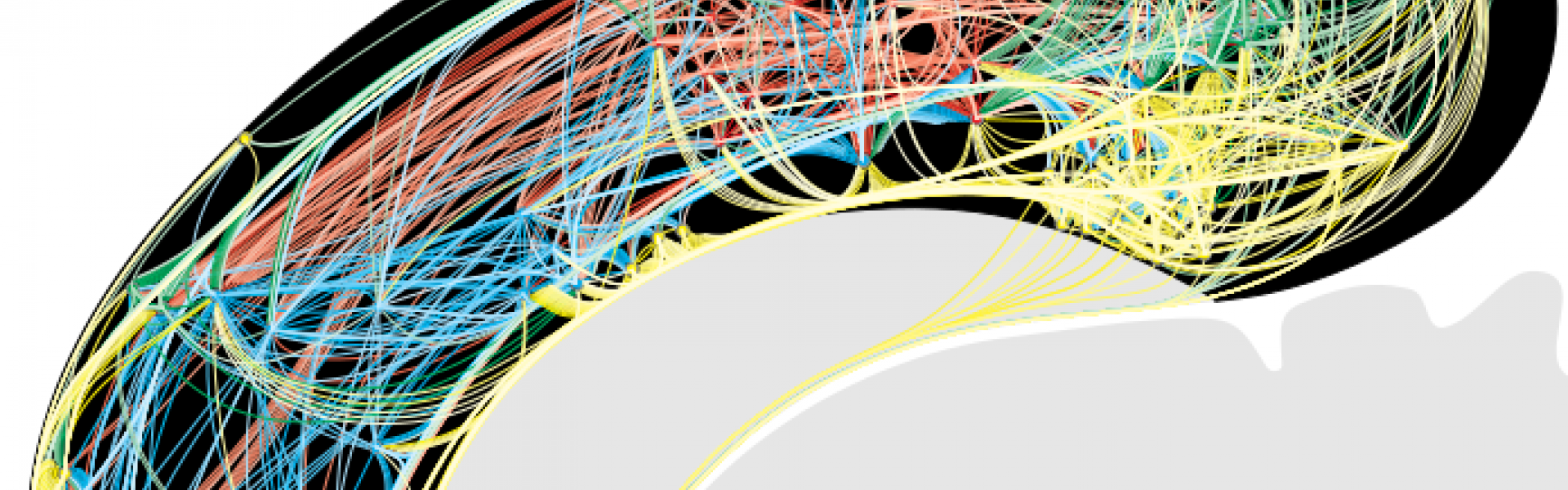
History and future of systems neuroscience
An interview with Professor Larry Swanson, University of Southern California, conducted by April Cashin-Garbutt, MA (Cantab)
‘Systems neuroscience needs a conceptual framework’ this was the overarching vision given by Professor Larry Swanson at his recent seminar at the Sainsbury Wellcome Centre, where he also gave the inaugural ‘Foundations in Neuroscience’ lecture on the history of neuroscience. In the following interview, Professor Swanson outlines the long quest to understand the basic wiring diagram of the nervous system and why systems neuroscience needs a conceptual framework.
Please can you give a brief history of efforts to understand the basic wiring diagram of the nervous system? How did you first get interested in this problem?
My own interest in this problem grew out of my research on what circuits are involved in behaviour. For the last 35 years I have been doing a lot of functional circuit tracing and as I thought more about how the brain controls behaviour, I realized that almost all behaviour involves the whole nervous system. And so, at some point, we need to understand the basic wiring diagram of the nervous system.
The nervous system is the last part of the body we really don’t understand or have a simple conceptual model for. Scientists know how the circulatory system, the digestive system, and breathing work, but when it comes to the nervous system, we don’t have a way of describing how this complex network works as a whole.
Why has it taken so much longer to understand the nervous system? Is it just because the nervous system is so complex?
The nervous system is much more complex – it is a network of billions of neurons wired together in a genetically programmed way. People have thought about how the brain works, ever since classical Greece and there are some very interesting theories that Aristotle and Galen came up with.
Even in ancient Greece people knew that we had sensory nerves for vision, smell and hearing, and that those nerves went into the brain. They also knew that the nerves somehow brought together information for thinking, imagination and memory and the product of this information would go down the spinal cord and along the nerves to produce behaviour. By the 17th century people started thinking about the grey matter and white matter of the brain and their role in behaviour and thinking.
Over the centuries we’ve obtained mountains of data about the brain, but there has been so much that we’re now under a tidal wave of information. And so the question now is how do we synthesize and think about all that information in a way that makes sense, how do we simplify it?
What new methods are being used to help make sense of all this information on the brain?
The new methods that have been applied in the last three, four, five years are basically modelling tools for complex information that are based on network theory and network analysis. Such general tools have been around for quite a while and apply to any complex system, not just the nervous system.
The tools started in sociology, where researchers were thinking about networks of people and connections between people, for example how people group into families and communities and countries and so on.
We’re starting to think about the brain and its complex network with the same basic tools. What are the basic hubs? What are the basic groupings of nerve cells at different levels of complexity? So it is really a new way of dealing with the complexity of the brain.
A lot of this work has been stimulated by computer science, where they are not afraid of big numbers. The internet is a gigantically complex thing that has really evolved just in the last 30 years. And so when neuroscientists talk about billions of neurons and so many systems, computer scientists are able to offer tools because they are used to dealing with such big numbers.
There has been a revolution in the last decade, producing a whole new way of thinking about the complexity of the brain. Just like the circulatory system, which is very complex anatomically, but is easier to think about through a simple model of a pump, the heart, with arteries coming out and veins coming in and capillaries communicating between the two. In the same way, we’d like to develop a simple model for the brain so that we can start to this about this complex system conceptually.
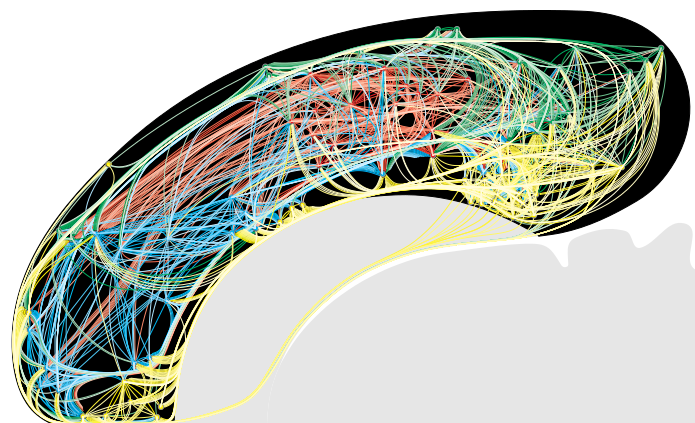
Why do we need a conceptual framework for systems neuroscience? Please can you give some examples of other conceptual models?
A conceptual model leads to hypotheses. For example, the double helix for DNA was actually a structural model when Crick and Watson proposed it in 1953, but once they had that conceptual structural model, it was the foundation for modern molecular biology because the logic of it was so clear and it led to the central dogma: DNA leads to RNA leads to proteins. The whole modern logic came out of having an accurate conceptual model.
The other great model is the Periodic Table of the elements, which was proposed in 1866. Although it was very incomplete at the time, the model had rows and columns and it predicted things that would be in those rows and columns that weren’t there, and the relationship between sets of elements.
In the same way, we’d like a conceptual model of the nervous system that would lead to testable hypotheses. Then we can go back and forth between a conceptual model and data and modify the model based on new data, and over time build up a better and better model that helps organize and think about all of the huge amount of data that’s coming through.
Ultimately, a conceptual framework would help us organize and think about the meaning of this gigantic tidal wave of data that’s coming in.
Please can you outline the high-level structure-function wiring diagram approach? How would better circuit diagrams help?
The idea is to break the nervous system down into different subsystems. As an analogy, think about the human body and the 10 different systems students learn about in medical school: the circulatory, digestive, respiratory system and so forth.
You can think about how each subsystem works, and of course they all interact, but it is a way of organizing your thinking about this really complex human body. Break it up into subsystems that you can deal with and specialize in.
We’d like to have something like this for the nervous system. For example, maybe there would be one subsystem for emotion, one for the sensory systems and so on. We’re not sure what the subsystems are going to be at this point, but basically we’re looking for a way of simplifying this very complicated structure into parts that you can understand more easily.
What progress has been made on assembling and analysing this wiring diagram for mammals?
The first real wiring diagram was done in C. elegans, a soil roundworm, based on electron microscopic analysis of the whole animal that allowed the creation of a map of every nerve cell and every synapse. This simple wiring diagram is still being worked on and new analysis methods are being developed.
Similar work is being done in the fruit fly, Drosophila melanogaster, and also the zebrafish, Danio rerio. Scientists are also beginning to look at developing a wiring diagram in mammals, such as mice and rats, and eventually, using different methodologies, they would like to develop a wiring diagram in non-human primates and humans.
The methodologies are developing incredibly fast right now, so there is a huge amount of progress being made in terms of gathering data. However, I believe less effort is actually going into thinking about what the data means.
How do we encourage people to go from ‘data gathering’ to ‘analysis’ mode?
This is difficult, because it is one of those things where until the answer’s there, you’re not sure you need it.
Take the human genome for example. There was a lot of controversy around the benefits of sequencing the human genome in the early stages. A lot of people thought it was essentially a waste of time or purely technical and there were a lot of arguments against doing it.
Now the human genome has turned out to be a wonderful thing and has had a tremendous impact on personalized medicine for example. However, until the results were actually in, it was hard to know the benefits. The project also gave us the boundary conditions for thinking about the chromosomes, for example, how many genes and transcription factors are there? This told us what the magnitude of the problem was.
I think understanding the wiring diagram of the brain is going to have the same basic benefits that the genome had for molecular biology. I think there will be ‘Connectome Wide Association Studies’ for mental diseases, just like there are ‘Genome Wide Association Studies’. Furthermore, I think, when those two come together, we’ll make huge progress on understanding how the brain works and what happens in disease.
In the last 50 years we’ve taken a reductionist approach to neuroscience and now we finally have the tools to start building it back up so we can understand how it works as a system.
How do you identify a potential hierarchy of subsystems and what tools do you use to achieve this?
Basically, this is a data driven approach, where data is organized into tables that can be analysed mathematically using formal network analysis. The tools are evolving constantly, just like the data, but essentially the tools look at systems and subsystems within systems.
As an analogy, think about airline routes. You can look at a map and see all the different airline routes around the world, but then you can break it down into what is going on in each country. You can ask questions like, what are the biggest airports? What are the biggest airports that are connected to each other? Then you can start to think about these networks and what would happen to airline routes around the world if Heathrow shut down for a week, for example. How would the closure of Heathrow reverberate through the whole system? Or what if you shut down two giant hubs at the same time, such as JFK and Heathrow, what effect would that have?
In the same way we can ask, what effect does a lesion in some part of the brain associated with Alzheimer’s disease have on the whole cognitive system? So you can start thinking about diseases like Alzheimer’s in terms of what parts of the brain network are actually affected and then how do those effects spread through the rest of the system?
Most people aren’t thinking in this way at the moment. They’re looking for one place where something went wrong, rather than thinking, how that affects the parts it is connected to. How does that spread through the system affecting gene expression, function, everything else?
Is it difficult to model brain plasticity?
You can actually model plasticity because you can look at different states, in the same way as you can have a gene expression chip at different stages of a disease. The point is that you need a map to begin with.
In essence, we are trying to create a Google Map of the brain. Firstly, we need to start with geography, that is, just where you are and how do you get from one place to another? And then you can start adding in restaurants, gas stations, any kind of metadata you want to map. Finally, you can build up a dynamic map, by adding traffic patterns, or in our case how the brain is operating in time. How is information flowing through the brain?
What are the main challenges that need to be overcome in terms of progressing further with this wiring diagram?
The main challenge is to figure out how to experimentally study a dynamic network within the brain. In other words, to see how parts of the brain are operating at the same time in a particular behaviour.
It is a massive challenge but technology is progressing really fast and the techniques are probably going to be worked out first in very small animals such as flies. However, how to apply those techniques to mammals, and ultimately to people, is another huge question. fMRI is a really interesting method, but it is extremely low resolution, so that’s a problem.
To me, probably the most important unsolved question is how to bridge the gap between human neuroscience and animal research. How do we apply the knowledge, from all the experimental work in animal models, to how the human brain works? Similarly, how do we use all the information we’re getting from functional imaging in humans, to understand the underlying mechanisms in animal models?
Essentially, how do we go back and forth to inform human research with animal research? How do we inform clinical and experimental research? This is a big problem because, right now, human neuroscience and experimental neuroscience are almost separate disciplines. They are not completely separate but there should be a lot more interaction between the two.
Part of the problem is that the two disciplines use different terms, but really they should have a common vocabulary. This may seem trivial, but it is a gigantic barrier.
How did clinical and experimental research become so separate?
I think a lot of this problem has to do with the benefits and faults of MRI brain imaging. The resolution is so low, whereas the work in animals is all done at the microscopic level and so it’s histology, it’s individual neurons. And you’re really talking about very specific parts of the brain that have been studied microscopically. In contrast, functional MRI refers to gross anatomy and involves gyri and lobes rather than the specific parts of the brain that people talk about in animals.
The cortical anatomy, the gross anatomy of human cortex, has completely different terminology than you’d use for the cortex of a mouse or a rat. The common vocabulary is microscopic, it’s histology, but that’s actually not done with fMRI. Thus the low resolution of human imaging at the moment is an impediment to transferring that information to what we know about rodents and vice versa.
Unfortunately, the incredible research that has been done on, for example, the mouse visual system, is not applicable to what we know about the human visual system. It doesn’t have to be that way, but at the moment it is. We basically have two different scientific cultures that are not making a big effort to bridge the gap.
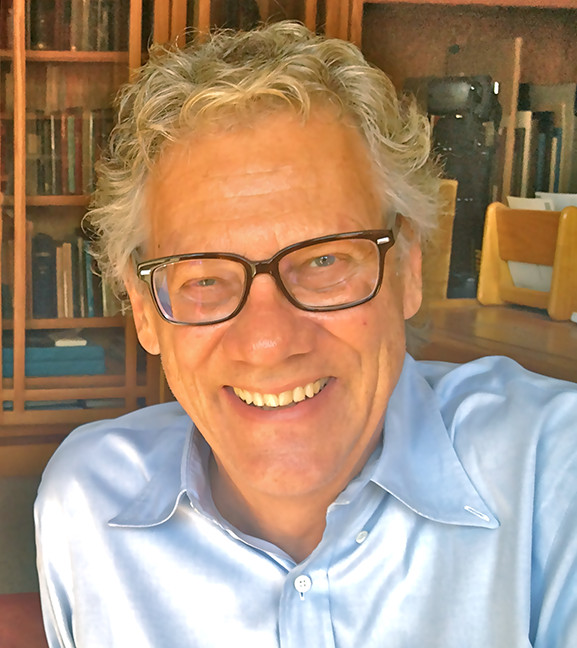
About Professor Larry Swanson
Dr. Swanson received his undergraduate degree in Chemistry from Pomona College (Claremont CA) and his PhD in Neurobiology from the Washington University School of Medicine (St. Louis MO), where he also did postdoctoral work with Max Cowan and Rita Levi-Montalcini. He then spent 10 years at the Salk Institute (La Jolla CA) before moving in 1990 to the Program in Neural, Informational, and Behavioral Sciences at the University of Southern California (Los Angeles). He is a member of the US National Academy of Sciences and a past president of the Society for Neuroscience. He is the author of Brain Architecture: Understanding the Basic Plan (OUP, 2003, 2012)