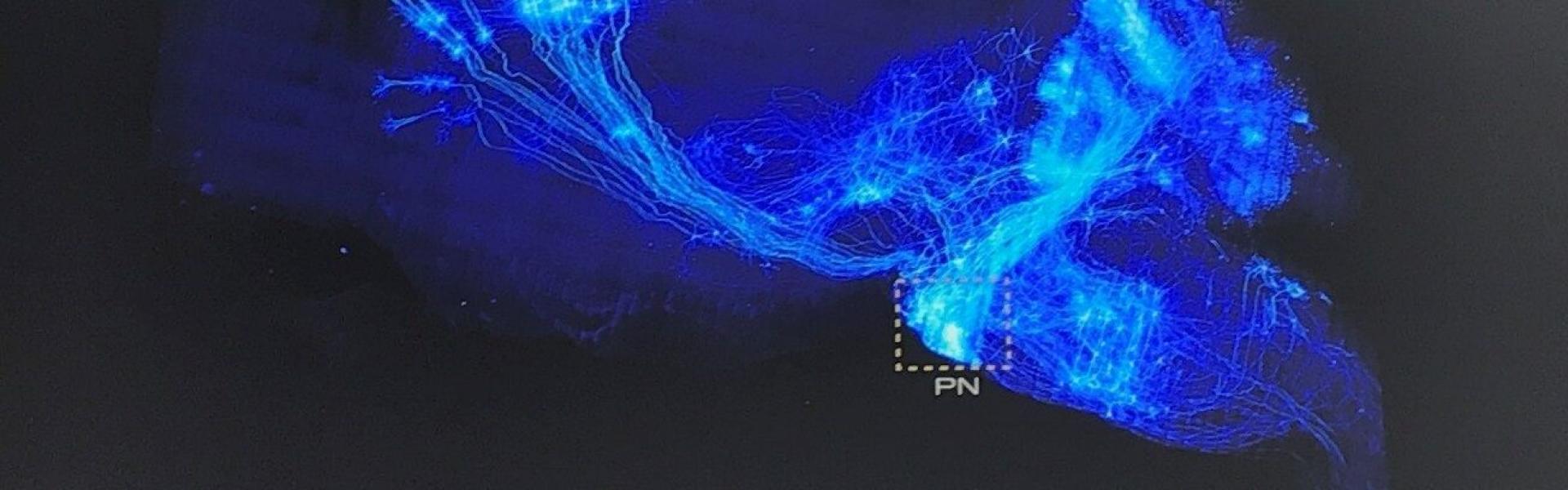
Neural circuits of dexterity
An interview with Dr Adam Hantman, Janelia, conducted by April Cashin-Garbutt, MA (Cantab)
Dexterous movements are at the pinnacle of motor control and are likely to be performed by a diverse set of neural circuits. In the following interview, Dr Adam Hantman outlines how his lab is attempting to identify and understand the neural elements responsible for dexterous motor control.
How do you define dexterous movements? How much of this activity is learned?
The definition of dexterous behaviour is quite controversial, but the way I define it is a motor behaviour that you can get skilled at, i.e. by practising you can achieve an increase in performance.
Classically dexterous behaviour has been thought of as a movement carried out by your hands, although lots of people argue that you can also do dexterous behaviours with other parts of the body. The important concept is that dexterous behaviour is a complex action that you get better with over time.
For example, writing is a dexterous behaviour. A two year old can move a pencil around the paper but in order to actually write, this is a skill that they have to achieve.
One of the things that we like about these dexterous behaviours is that because they require learning, and because they are a complex operation of the brain, they involve large swaths of the brain. This makes it interesting if you want to understand how brain dynamics work, where you are looking more globally than in one particular area of the brain.
How much of this activity is learned? By definition, dexterous behaviours have to be learned. The basic operations probably sit somewhere more an elemental position in the brain where you don’t have to learn them, but in order to get to dexterous, it’s almost by definition is has to be learned.
Now where that learning occurs in the brain is still up for debate, and it probably involves many places in the brain, but there is some evidence that dexterous motor behaviour critically involves motor cortex. One of the best indications of that is if you have a stroke in motor cortex, your abilities to move your hands are one of the primary things that maintain an effect. So cortex, we think is important, although I think that does not mean that other places aren’t important for learning. I certainly think that places like the striatum and the cerebellum have important learning changes to deal with the fine aspects of dexterous movement.
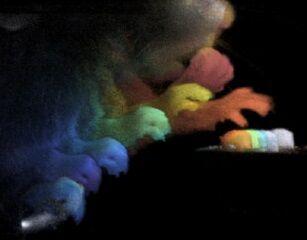
Where do you draw the line between what is dexterous movement and what is not? Is picking up a glass of water and bringing it to your mouth a dexterous behaviour?
Yes picking up a glass of water is definitely on the dexterous side as it involves fine control of your hand and it is certainly learned and you can achieve high skill at it. There have been studies that have documented such learning in babies acquiring the skill of picking up food and bringing it to their mouth.
In terms of where to draw the line, it is difficult. One complication is that in science we want to use a behaviour that we can do hundreds of trials with, but in real life dexterous behaviour is wildly adaptive as we use our hands all the time not in a practised movement, but when interacting with the world. In order to do that we had to learn lots of features about the world and about our arm.
Grooming is a behaviour that looks very dexterous but is more innate. Although grooming involves using your hands, and you are certainly interacting with your body in a very specific way that looks like a dexterous behaviour, it seems like this behaviour is more. I’m not saying grooming is not dexterous, but these are behaviours that the nervous system seems to be able to do largely on its own.
What operations are needed to generate and maintain dexterous behaviour?
Like any motor action, many operations are important in generating and maintaining dexterous behaviours. You need to have learning processes and feedback but most importantly you need to have a model of the world and also a model of your arm.
The complicated operation of the brain is to have those two models interact to figure out how your arm, with all its degrees of freedom, can interact more efficiently and productively with an object in the world.
We don’t really know how the brain does this, as how you represent a model in general is quite complicated. The model does not sit in just one area of the brain, it is a brain wide network.
What techniques do you use to identify the neural elements responsible for dexterous motor control?
We’re a little bit of an unbounded lab in that we will do whatever technique it takes! One of our main techniques is electrophysiology with perturbations. We record from what we think are key nodes in the circuit and we sometimes restrict ourselves to the bottlenecks that we think information really has to funnel down through so that our recordings are efficient for that area.
We like to understand the neural dynamics during a behaviour with and without another area of the brain and then try to relate the firing patterns, the perturbations and the behaviour all together. We also try to ground ourselves in anatomical substrates and so we do a lot of anatomy using viral tools and use whole brain microscopy techniques.
We also do a lot of transcriptomics in the lab where we try to uncover the molecules which give cells their identities and use that information, not only manipulate specified parts of the brain but also, to try to understand the computations that are involved and how they’re pulling off those computations given their properties.
We also do some imaging when we want to study things that evolve over a longer timescale. For example, we are just starting to get into studying the learning of tasks, and imaging allows us to monitor the circuits over time. Although you give up your fine temporal resolution, you get this extended temporal view of what’s going on.
In essence, we are a question driven lab and if there’s a technique we don’t know, we just go figure it out!
What types of experiments have provided the most insight into understanding the circuit basis for dexterous behaviour?
The perturbation recording experiments have provided the most insight for us. The combination of perturbations and recording have really helped us to try to understand what the neural dynamics are, which parts of the neural dynamics are really important and how, in this complicated neural space, these neural dynamics are being assembled from the various bits of the brain. That kind of dissection of the neural dynamic patterns is very difficult to do by inference but you get a lot of insight by perturbation if you can monitor it during the perturbations.
This is one of the fantastic things about being a neuroscientist right now. Before you would do a classic lesion somewhere and you would study the behaviour, or you would identify a beautiful behaviour and then record, and now we can combine those two things. We can do a perturbation on random intermittent trials while recording and just look at this on a trial by trial basis. That ability to combine these two grand techniques in science has really only been possible since the advent of optogenetics.
We are just starting to learn to combine those two things but it’s basically an unprecedented position to be in and I think we’re starting to see the fruits of it now but we’re just at the beginning. I definitely am most excited about that right now.
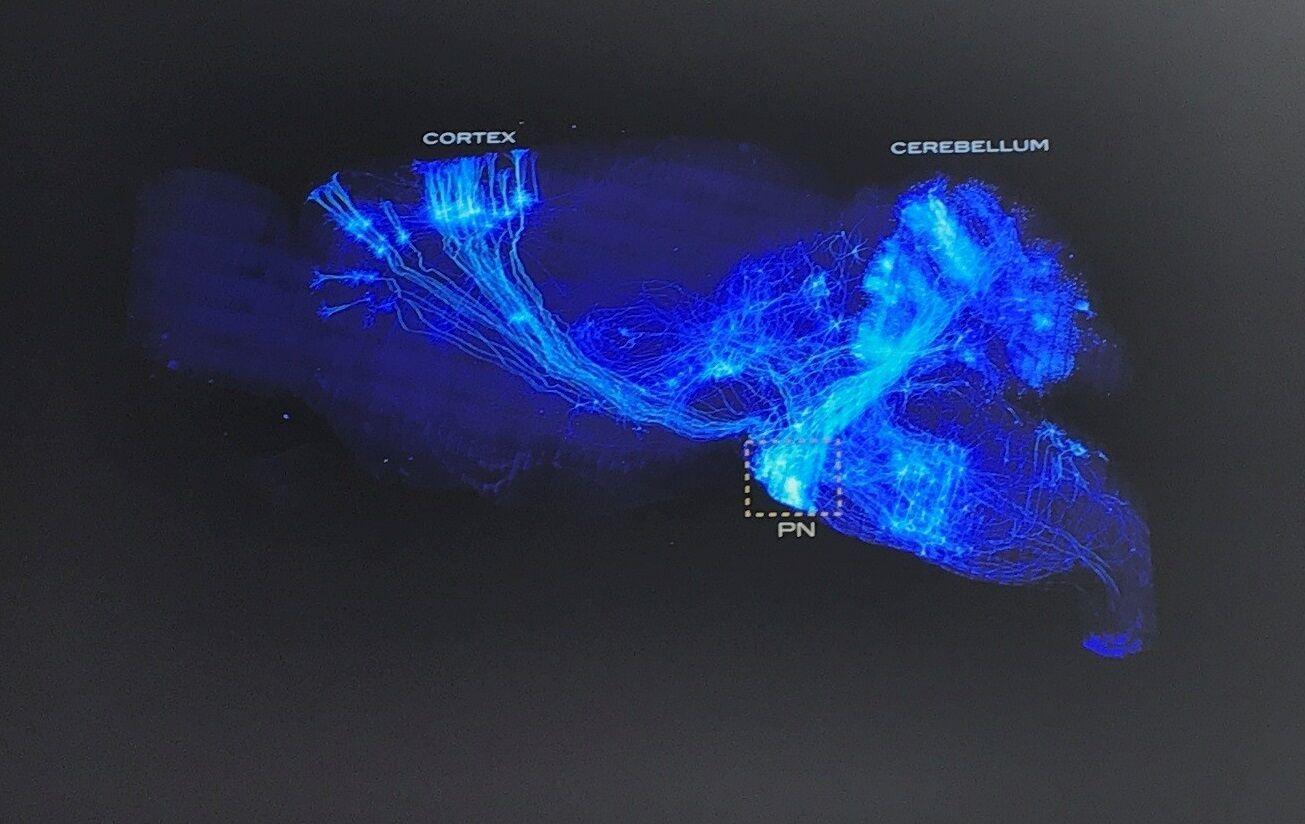
Can you please outline your current research on the role of the cortico-cerebellar loop in a skilled reach-grab-eat task?
The current research that we’ve done is looking at understanding one loop in the brain. The loop we have been trying to understand is the cortico-cerebellar loop and the reason we chose this is because the cerebellum has a really good potential to hold the model of how the arm works and the cortex might have some information about the plan and what the goal was. And so, if we want to understand how these models interact, we thought this was a really interesting loop to study.
We have been working our way through the loop recording and perturbing at all the stations we can, which is not all of them, and we focused on what the role of the cortex is, which has been very controversial over the last 5 – 10 years, and what this downstream area of the cortex, called the pontine grey, does with the cortical information and what this transfer of this information to the cerebellum does.
The one bit we haven’t done is how the cerebellum feeds back to cortex, this goes through thalamus and we really lack the toolbox for cracking open that part of the circuit. We had to take a step back and crack the genetic code in thalamus in order to make the tools that would allow us to do selective perturbations so that’s one of the things we’re doing next.
We’ve been recording and manipulating, doing fine scale anatomy across this loop, and trying to understand every part of it, but of course, this is just one of the loops in the brain. Now we’re starting to think that we should take on other major loops in the brain, not only to understand them in isolation, but I think the real power is going to be understanding how you put all this information together.
The loops all have these convergent spots, for example, the cortex has the cortico-cerebellum loop and also the cortico-basal ganglia loop and both of them are going to hit right back on cortex. They both look like they’re holding different pieces of information doing slightly different operations. What you get from each one of these loops and how does this information from the rest of the brain modify and shape the neural dynamics in the cortex that seem to be so critical?
How many loops are there thought to be in the brain?
It depends on how you define loops. There is probably an internal loop within cortex and then next shortest loop is probably the cortico-thalamic loop. Then there’s the cortico-basal ganglia loop and the cortico-cerebellar loop. There’s the cortico-reticular loop and the cortico-spinal loop. There is also this loop in the world and so you have all these little nested loops and the smallest loop is probably a loop within cortex and the biggest loop is the one that goes through your body.
Basically at almost every other station you have these other nested loops and this is the complexity of the brain. We have all these loops, they’re probably all doing slightly different operations, but they’re also all being combined. How are the combinations being pulled off in a rational way? What do you use each one of the loops for? These are tough problems.
The problem with the cortico-body loop is it is slow and it has all of this noise about the motor output and the sensory world. We think one of the reasons why you have these internal loops is for the brain to make predictions about what’s going to happen so it doesn’t have to wait for it to actually happen. We think that’s not only important for things like planning but also for corrections as we can make comparisons between our expectation of the world and something that actually happened.
What is the next piece of the puzzle your research is going to focus on?
The next piece of the puzzle is the integration with other loops. One of the things about motor control that no one wants to talk about is the huge hole in our understanding about the spinal cord. And so we are going to try to understand how a cortical message is received by the spinal cord as I think that is a major question that someone needs to answer.
Right now we’ve only studied the execution of a well learned action, but how the animal learns this action is really interesting to us so we are going to start thinking about the learning and adaptation of this behaviour in a couple different contexts.
The last thing that we are getting very excited about is understanding this basic operation of reaching out in the world, interacting with it and manipulating the world. We have some understanding of the basic circuitry for rote memory in an animal, but now we can start adding more cognitive aspects to the task and ask what parts of the brain are recruited when these more cognitive aspects are added.
What if I have multiple choices in the world that I want to interact with? How do I decide between one motor program and another motor program? Or what if I actually have no idea what the world is and I have to sense what the world looks like and then convert that sensory information into a motor command i.e. perform a real coordinate transform from sensory to motor? That’s a basic operation of the brain and there’s been lots of great work in the primate but putting that down to a mouse where we can actually do these more modern manipulation techniques, I think, would be very exciting.
There are other things that are more on the predictive side, if I have a task where I have to predict the outcome of step one in order to perform step two, how do I make those predictions so I can perform the task at a high level? Adding those cognitive aspects onto this basic operation, I think we’re almost into a position where we’re well suited, where we understand the base and we can see how you add parts onto it.
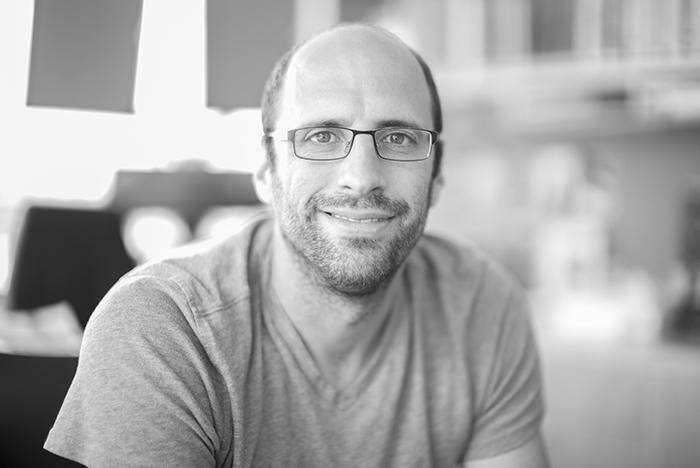