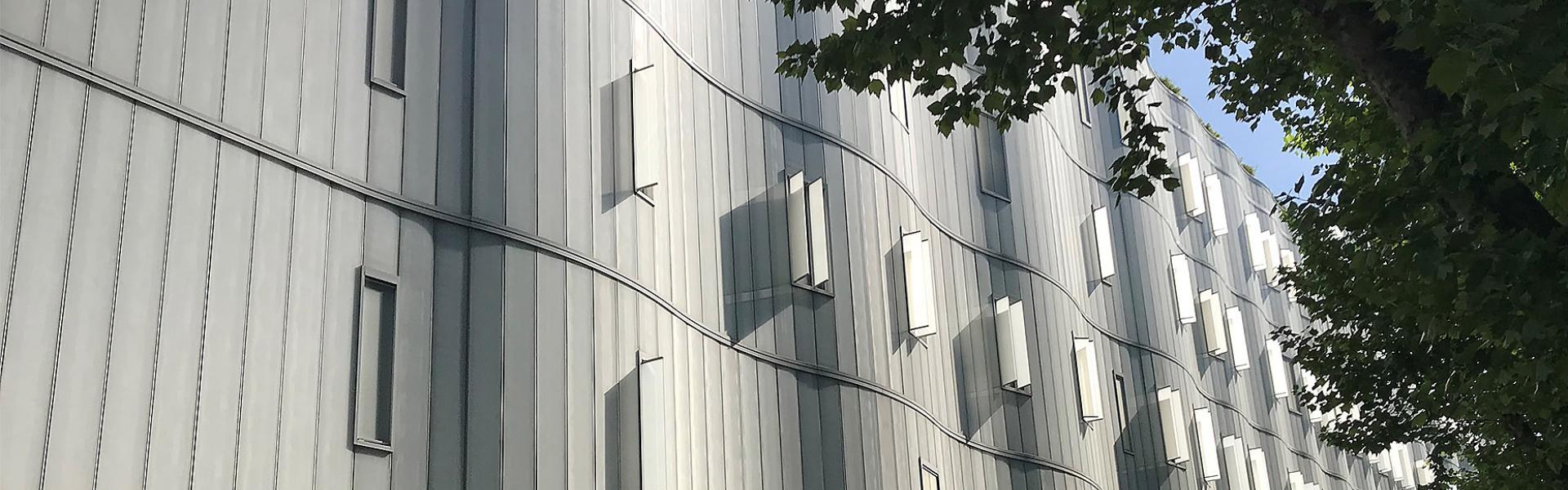
Selective attention: what is the parts list?
An interview with Professor Rich Krauzlis conducted by April Cashin-Garbutt, MA (Cantab)
What is the “parts list” for selective attention? That was the question Professor Rich Krauzlis opened with in his recent SWC Seminar. Through an amusing analogy to the components left over after building an item of flat pack furniture, Professor Krauzlis explained that despite knowing a lot about parts of the brain involved in selective attention, there are still areas we don’t fully understand, and particularly the question of where they fit in. In the following interview, Professor Krauzlis’ outlines his research findings to date in this field.
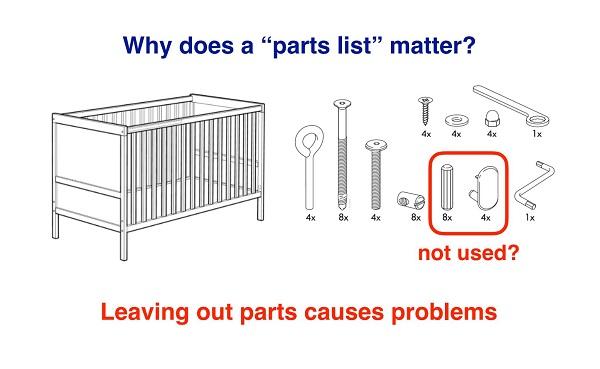
How do you define selective attention?
Selective attention is a particular aspect of attention that involves processing a subset of the available sensory inputs, either to guide an action or even for things like perception or thinking. It is ‘selective’ because, depending on the context, the subset is appropriately chosen so that it matches the goals of the animal. For example, under one circumstance you may choose a particular visual or auditory signal as the thing you are processing, but if your goals change, even though you may have the same inputs, what you process changes.
It is useful to distinguish selective attention from other aspects of attention like arousal and vigilance. Arousal and vigilance are related to your overall motivation or engagement. For example, an air traffic controller needs to retain a certain level of vigilance and alertness in order to do their job properly.
It is likely that these types of attention are functionally nested as it is hard to properly apply selective attention if you are not vigilant, for example if your mind is wandering. However, it is possible for you to be vigilant but not selectively attending.
In some sense you have to actively exclude things. People emphasize the improvement or enhancement of things that you are attending to, but it is at a cost as you have to actively ignore other things. If the knowledge you are applying to guide your selective attention is inappropriate, that can make you miss things. That’s why a naïve observer with a beginner’s mind can notice things that an expert might not see, as an expert knows what to ignore.
Which brain regions has selective attention traditionally been attributed to?
Selective attention has largely been attributed to sensory cortex. In visual attention, it is largely visual areas. The effects become larger as you move along the hierarchy, so the effects in primary visual cortex (V1) are smaller than they are in later visual areas.
Most work has been done in later stages of visual processing, such as area V4, where the neurons respond to orientation and colour, and middle temporal area (MT), which is specialised for processing motion. These areas are where most of the effects have been documented. Studies have shown that depending on the task condition, neurons will increase their firing rates or be more selective, even though the visual stimulus has not changed.
It is thought that the process by which the activity of these neurons is enhanced is guided by signals that come from frontal cortex and parietal cortex, so these areas are viewed as control regions for determining how you are allocating attention.
Can you please give an overview of your previous work on the role of the superior colliculus in controlling selective attention? What was surprising about this research?
The superior colliculus (SC) was known to be important for orienting, in particular for movements of the eyes and head, and there was work showing that even if you don’t physically move the SC is also important for orienting internally, such as orienting attention.
Other labs had done activation studies, where they stimulated electrically and showed that the animals behaved as though they had shifted their attention. We did the converse and suppressed activity and showed that the animal had difficulty attending to that part of space.
Conveniently, the SC contains a nicely organised map of the visual world, so that if you suppress activity locally within the SC you affect a specific portion of the visual field and the animal has difficulty making judgements about the stimulus when it’s placed there. However, they are not blind, so they can still see the stimulus.
To show this, you can give the animal just one stimulus to judge and they can do this about as well as a normal animal, but when you put competing stimuli in the visual field, then their performance is severely degraded. This is similar to what you see in clinical cases of human neglect, where patients tend not to notice or engage with things in their environment that are in the affected side of space.
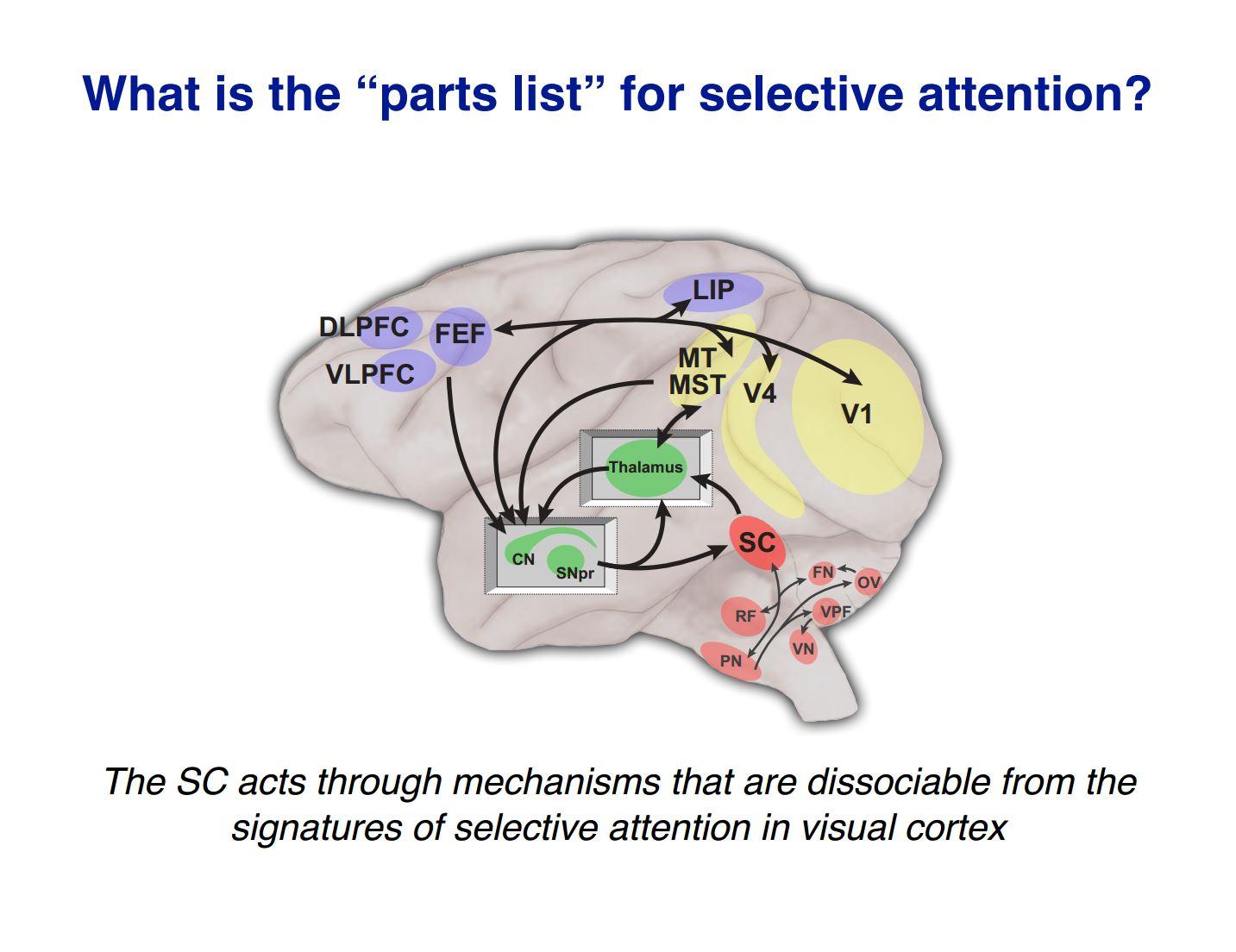
What was surprising was that the SC was thought to be another source of these controlling signals that regulate what’s happening in visual cortex, but when we checked, by recording from neurons in the visual cortex as we did these manipulations, they didn’t change at all. The neurons in visual cortex showed all the normal signatures of attention, even though behaviourally the animal had an attention deficit because of our manipulation in the SC. So whatever the SC is doing, these results indicate that it is not doing it through this standard, well-understood mechanism of changing how signals are represented in visual cortex.
The alternative idea was that you’re not changing what is represented but you are changing what gets used, so then we went on a hunt to find out where does that happen?
How are you trying to understand these counter-intuitive results?
We did a series of experiments using functional imaging to identify areas of the brain that were modulated as the animal was doing an attention task. Then we did the same manipulation of reversibly focally inactivating the SC and then see what parts of the brain changed. The results showed some things we expected from what was in the literature, such as changes in cortical areas like frontal cortex, parietal cortex and sensory cortex, but we also found a spot in the brain that was totally unexpected.
We also used transgenic mice so we had genetic tools to target specific populations of neuron. As we had hypothesised there may be circuits through the basal ganglia that might be important, as this area is a set of subcortical nuclei in the forebrain that’s important for learning what actions you should engage with in a particular context.
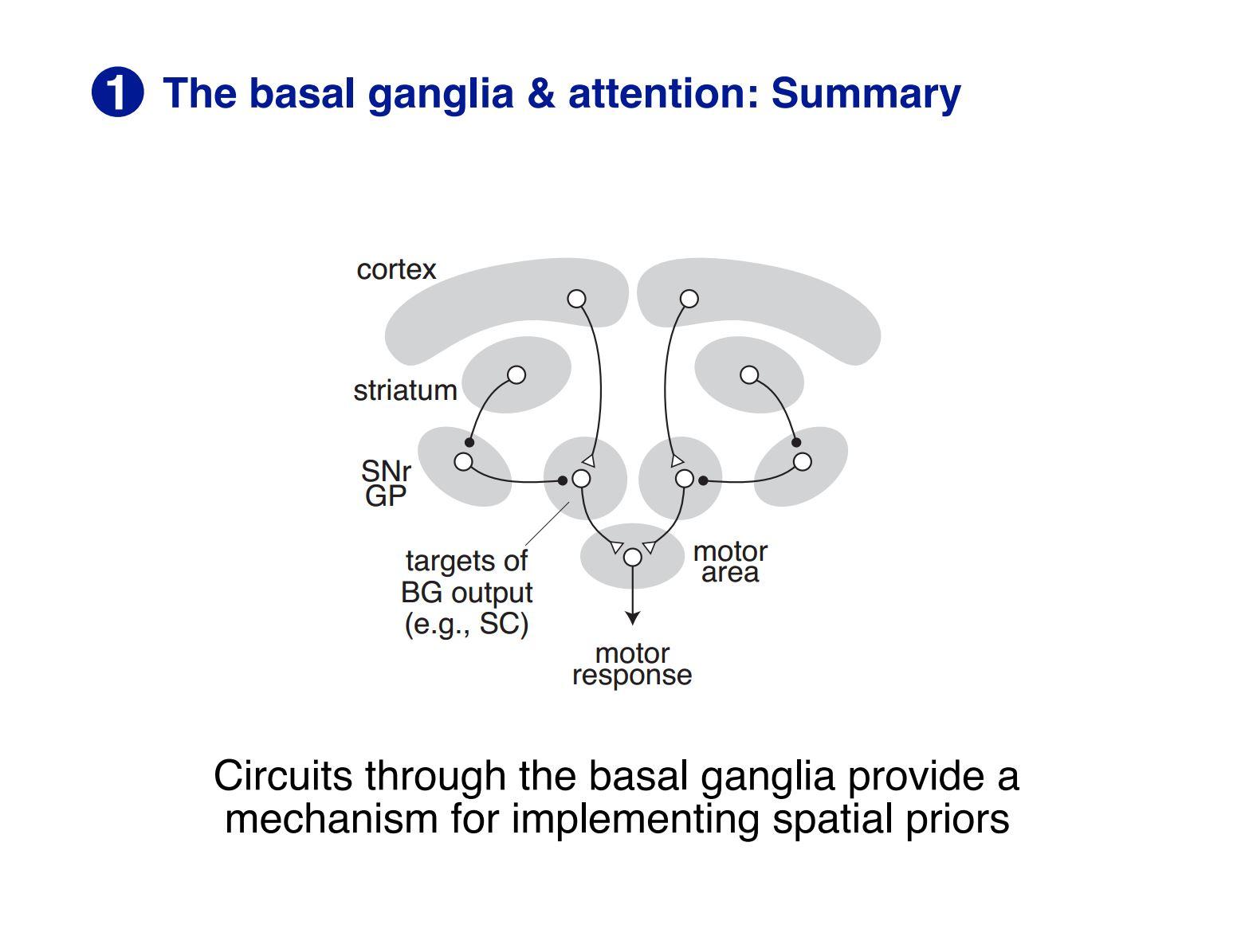
Do your findings indicate that selective attention arose as a result of the emergence of the neocortex or does the evidence point to selective attention being made up of several functions that were accrued over time?
This is an interesting thought experiment as you really can’t do an experiment about evolution, but we can speculate. An attractive hypothesis is the way selective attention in humans evolved was by starting as control over orienting. Basically, what we are trying to do in our behaviour is extract value from the environment, such as getting food, finding mates and avoiding danger. In each case, you are trying to make sure your actions are consistent with the constraints of your environment and your internal needs, so that moment by moment you are extracting value.
In lots of animals, such as fish, frogs and so forth, there are mechanisms that are completely subcortical and seem to accomplish much of this. For example, a frog’s behaviour can be split into two main classes, there are things in the world that they will detect and approach to try and eat, and there are other things that they will run away from, or freeze. In the simplest sense, just based upon the visual parameters, if it is a small horizontal thing in the lower visual field that is moving relatively slowly, a frog might think of it as food; if it is moving in the upper visual field, if it is small they might snap at it, but if it is large, and particularly if it is looming, then it is dangerous.
There are looming detectors, for example, in these subcortical circuits in the frog including in the tectum, the structure on the roof of the mid-brain. Some of these areas are even present in mammals. For example, one of the popular paradigms in mice is to have a looming stimulus presented on top of a cage with a mouse. The mouse will be happily walking along in the cage until the looming stimulus, created by the black dot on the flat panel display getting bigger and bigger, presents and the mouse will run into a shelter, so there is very strong escape behaviour that you can elicit.
There are a class of things like this, where based upon their visual properties and where they are located in space, you can fairly reliably judge what the value of it is. Big things looming in the upper visual field are dangerous, and small squiggly things in the lower visual field can be things you might want to explore and possibly try to eat. Making those sorts of choices, is something very basic, but this sort of selective orientating may be the underpinning of how we do selective attention.
Compared with selective attention, selective orienting is clearly reduced in terms of the number of things you can interact with and the types of responses you can have. One of the things you gain by having an enormous expansion of neocortex, like we have, is the sheer number of things you can recognise and the diversity of behavioural responses you can generate is enormously expanded. Then there are things like episodic memory, where based on a single exposure, you can remember a particular instance of something and then apply that as well.
The thought is that you start with something subcortical that involves circuits through the midbrain, thalamus and basal ganglia, which are parts of the brain that are present in all vertebrate animals. There are also the precursors of the neocortex, as some of the same cell types are present in those animals as well but they are not organised into a neocortex.
Something really interesting happens when the brain changes so that it has a large neocortex. It provided some competitive advantage to animals in being able to classify objects with more precision, so you can tell the difference between things. This was particularly important for animals that are social, so they can tell the difference between individuals.
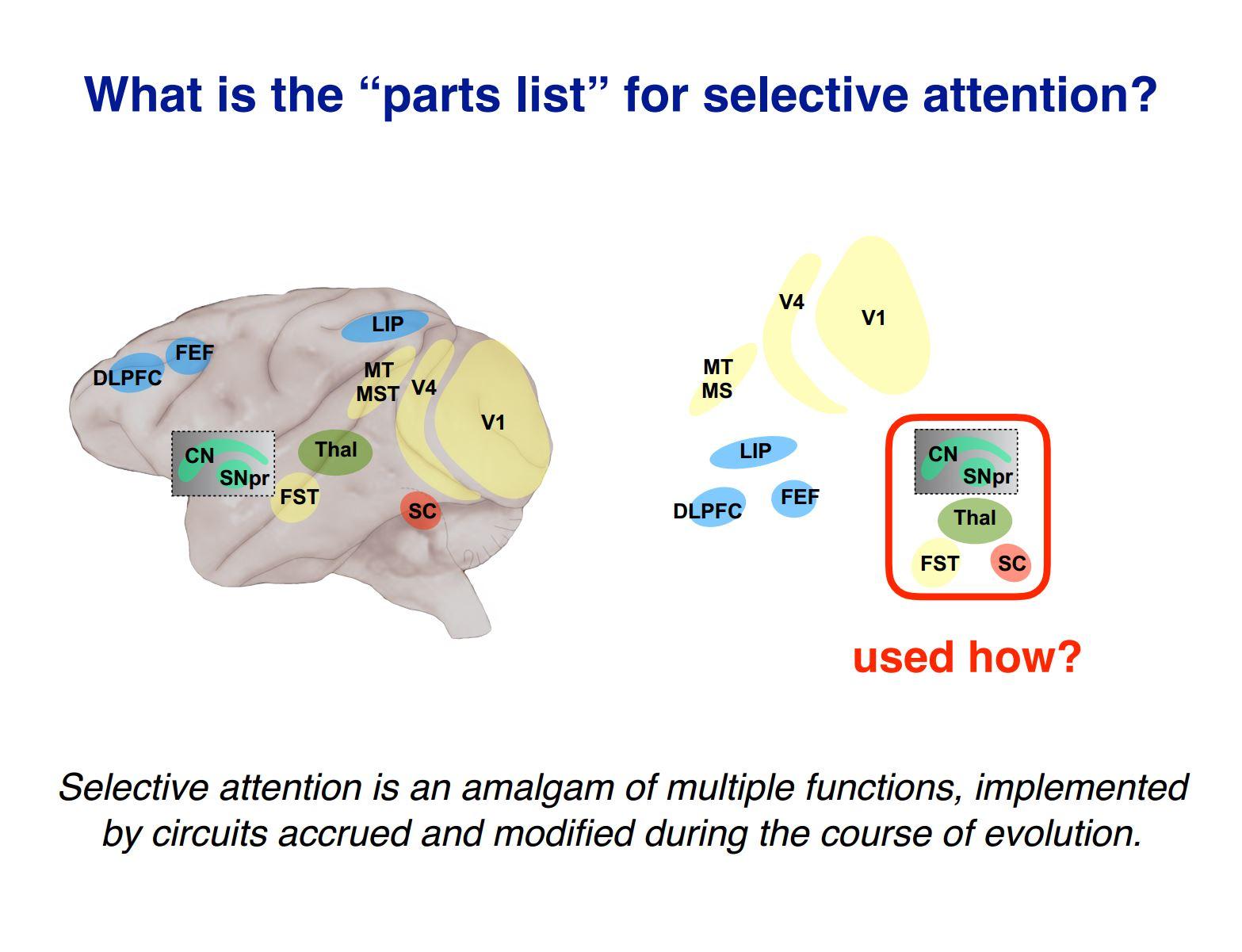
What can we learn from determining how older subcortical circuits interact with the more recently evolved components in the neocortex?
One question is in normal behaviour, which parts are most crucial? Cortex definitely is important, but I think we simply don’t know in normal behaviour whether it is the cortical processing that is normally the limiting factor or whether it is the subcortical components.
Based on the experiments we have done, it is much easier to cause behavioural problems by interfering with the midbrain than doing anything in most places in cortex (there is, however, a spot in the temporal lobe that is very sensitive).
We’ve done experiments where we interfere with the frontal eye fields and they cause behavioural impairments, attention deficits, but it is harder to get effects as large as if you interfere with the midbrain. The general issue is how dependent is this function on different parts of the brain, and it seems like selective attention is really sensitive to the contribution from subcortical areas.
Something else we are also interested in is understanding what each part contributes. For example, the area we have identified in the temporal lobe and its possible link to object recognition, might provide a way experimentally to get at this issue of what actually did happen during evolution and how do you go from an animal that is relatively fixed in what they can detect and how they can respond to animals like us that have enormous capacity for recognising individual instances of things and behaving in very flexible ways.
I’m hopeful that we’ll be able to understand the circuits so we can understand what the neocortex is really adding. Then comparing mice to primates is going to be very interesting as there are prefrontal cortical areas that primates have that mice don’t, also organisation of the sensory cortex is substantially different.
There are probably aspects of the way the neocortex is organised and how it interacts with subcortical structures that are responsible for us being human. There is something about the way our cognition is different from a mouse’s cognition. Humans are visually dominant, and so we, like a lot people, are studying vision in mice, but vision isn’t necessarily the dominant sense for a mouse.
The way a mouse thinks is probably substantially different to the way we think, because we often think visually and also through language. I imagine mice might think visually, but they also might use somatosensory senses, they might smell thoughts, or use touch. Wrapping our heads around what that would be like is important.
Will this work have clinical implications, such as enabling us to understand the causes of attention disorders or other psychiatric conditions?
This work does have clinical implications and that is one of the challenges, as with a lot of basic research, of how to effectively make that translation from basic research to something that can be clinically applied.
The direct implication is that looking at circuits that are subcortical identifies a set of neuronal targets outside of neocortex that could play crucial roles in disorders of attention. For example, when people have attention disorders, such as Attention Deficit Hyperactivity Disorder (ADHD), the sorts of behavioural problems they have, are not exactly what you would expect if you think the main mechanism for attention is regulating the quality of visual information. An individual with ADHD doesn’t have a particular problem with seeing or judging stimuli, what they have is more of a behavioural problem of remaining consistent in whatever behavioural goal they are trying to achieve and ignoring distractions.
The behavioural problems that people show when they have attention disorders are more consistent with the control of actions, which is one of the functions we understand structures like the basal ganglia to be involved in. So I think understanding what the basal ganglia and these other subcortical structures are doing in these selective attention tasks will put us closer to understanding what happens in a human with ADHD.
The other condition our work touches on is neglect. The behavioural effects we have when we suppress activity in the midbrain, or this novel area in the temporal lobe, look a lot like neglect. Neglect is a really interesting clinical syndrome that affects lots of people that have had a stroke, sometimes briefly but sometimes long-term, where they tend to ignore things in space.
Neglect shows up in lots of different complex forms, it can be centred on the visual field but it can also be centred on outside space. For example, regardless of where they are looking, they might ignore half of space. It can also be object-centred, for example when they eat off a plate they tend not to eat the food on one side. If they are in a clinical ward and then go out and come back to their room, rather than taking the shortest pathway, such as taking a single left turn, they’ll make three right turns instead.
Neglect is interesting because it is such a profound impairment and the patients are often unaware of it and it involves a variety of reference frames. In some people neglect seems to be linked to the eye, in others it is linked to the organisation of space, and in some patients it is linked to the organisation of objects, so it moves with the object.
By identifying the spot in temporal lobe, I think it is a possible animal model for trying to understand what is happening in neglect in people.
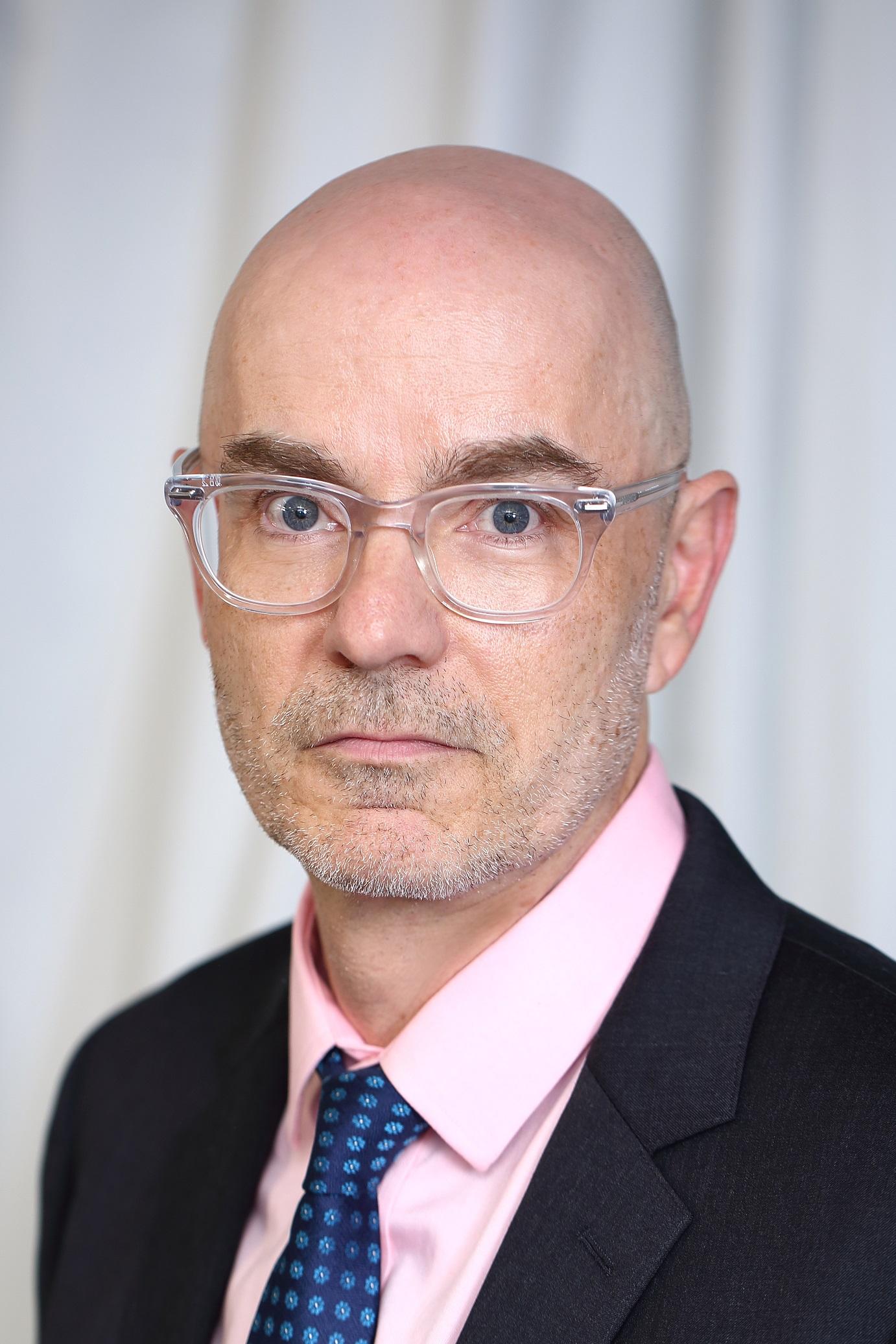
About Professor Rich Krauzlis
Rich Krauzlis earned his undergraduate degree from Princeton University and doctorate in Neuroscience from the University of California, San Francisco, in Steve Lisberger’s laboratory. After postdoctoral training with Fred Miles and Bob Wurtz at the National Eye Institute, he was recruited to the Salk Institute in 1997, where he was promoted to Full Professor in the Systems Neurobiology Laboratory. In 2011, Rich returned to the National Eye Institute as a Senior Investigator in the Laboratory of Sensorimotor Research and Chief of the section on Eye Movements and Visual Selection.
Work in Rich’s laboratory is aimed at understanding the brain mechanisms that link motor control to sensory and cognitive processing, using a variety of techniques to manipulate and monitor neural activity. Rich’s vita includes papers on pursuit and saccadic eye movements, physiological studies of the superior colliculus, cerebellum, and cerebral cortex, psychophysical studies of visual motion perception and visual attention, and computational modeling. His recent work has focused on the problem of how evolutionarily older subcortical structures interact with new cortical areas during visual selection attention and perceptual decision-making.