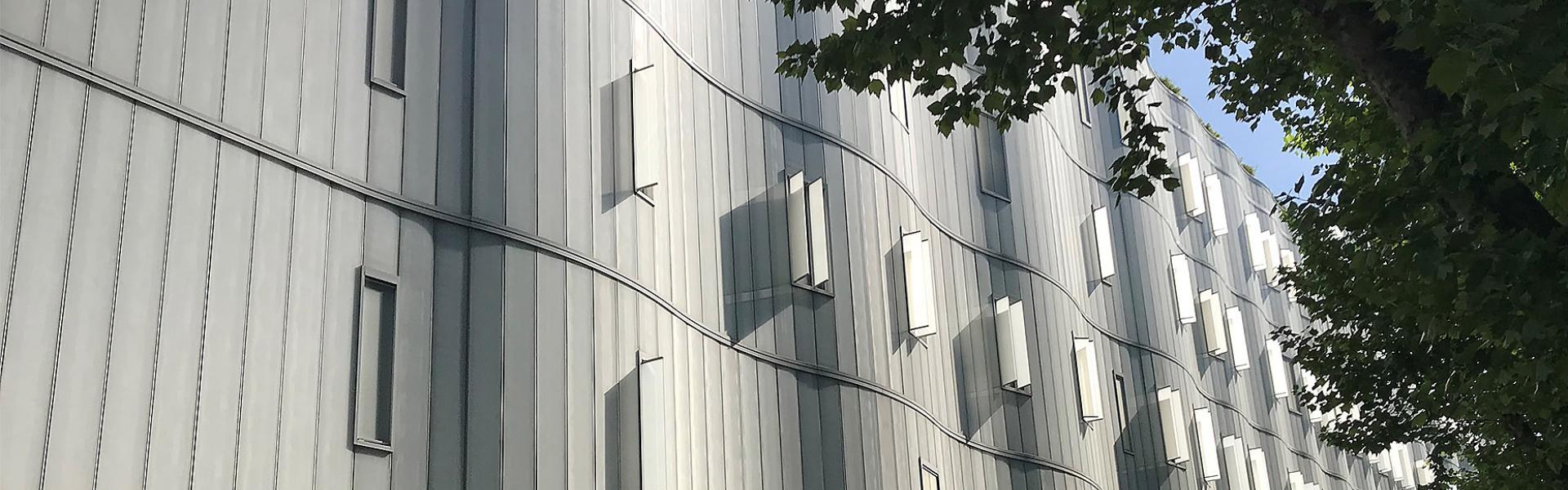
Towards a canonical computation in basal ganglia for the vigorous pursuit of reward
An interview with Dr Josh Dudman conducted by April Cashin-Garbutt, MA (Cantab)
Dr Josh Dudman, Group Leader at Janelia Research Campus of the Howard Hughes Medical Institute, recently visited the Sainsbury Wellcome Centre to give a talk titled ‘Towards a canonical computation in basal ganglia for the vigorous pursuit of reward.’ In the following interview Dr Dudman outlines his research on purposive behaviour and the techniques his lab use to try to understand the neural circuits underlying learning.
Can you please give some examples of purposive behaviour?
In my lab, the types of behaviours we are particularly interested in are purposive behaviours. This term was coined by Edward Tolman in 1932 who wanted to push back on the commonly held view at the time that animals only had passive stimulus-response behaviours.
Tolman was very interested in the idea that animals, like us, have, to some extent, deliberative decisions and notions about what they’re going to do, and that they perform actions with some intended goal. Driving to work was one of the examples Tolman gave in humans, but other examples of purposive behaviour include animals learning to press levers in exchange for a reward; or navigating through a maze to try to get to a goal; and waiting for something that they believe is about to occur.
How do animals learn to perform such behaviours?
One line of thought is that animals initially learn the relationship of their actions to the expected outcomes. There are still a lot of questions about how animals learn this and a common perspective is that they do a form of reasoning about the expected quality, or value, or how desired the outcome is. An influential model is learning through trial and error: you try a bunch of things, you see how good the outcomes are and you learn from them.
What techniques are you using to try to understand the neural circuits in the brain that mediate this form of learning?
A lot of our interest is in how animals control their own actions, or make decisions on relatively fast timescales. We focus on measuring activity in the brain at timescales of around tens of milliseconds and so we essentially use any approach that enables us to do this.
Sometimes, that involves using microscopy, or other optical techniques, to measure reporters of activities such as fluorescent molecules, which are engineered to give us an idea of when cells are active. We also focus a lot on electrophysiological techniques, which use electrodes of various sorts to directly measure electrical potentials within and between neurons.
We also like to be able to perturb the function of the brain, so we use a technique called optogenetics, which has been developed over the last decade. Optogenetics allows us to use light to rapidly modulate, or control, the activity of specific types of cells in the brain. Some of our work involves trying to develop techniques for optogenetics, such as making molecular reagents.
In addition, we are trying to design ways to do closed loop experiments, where we allow the behaviour of the animal to trigger stimulation within its brain. These closed loop techniques have been around for a long time, as a way of doing psychophysics, and understanding behaviour. Psychophysics aims at a quantitative understanding of behaviour. We’ve been trying to take advantage of the fast timescale of optogenetics and build systems that allow us to do closed loop interactions with just a few milliseconds latency.
Why are you focusing on the basal ganglia?
One of the reasons I first got interested in the basal ganglia was from work carried out in part by SWC’s Marcus Stephenson-Jones when he was in Sten Grillner’s lab. The last common ancestor of all vertebrates that we can infer was about 560 million years ago. As far as we can tell, they had a basal ganglia that was essentially the same as the one that all mammals (including primates) still have today.
To me, it is fascinating that natural selection maintained the basic architecture of this circuitry, even though the brain has undergone such massive diversification, especially through the mammalian lineage.
We’ve evolved this giant neocortex that has lots of new cell types in it, and things that are at least difficult to date to find in vertebrate ancestors, and yet as far as we can tell, the basal ganglia does not undergo very much innovation through all of that evolution. It basically just scales with the size of the brain that it’s found in. This makes me wonder what the utility is of the circuit? It hints at some relatively simple function that we might be able to figure it out.
In addition, there are a large number of diseases and disorders that have pathology within the basal ganglia. The primary pathology of Parkinson’s disease and Huntington’s disease, which are the second and third most common degenerative movement disorders, is in the basal ganglia. We also think lots of other disorders, like Obsessive Compulsive Disorder (OCD) and tic disorders, have pathology in the basal ganglia. We’ve been really interested to see whether additional basic science understanding of basal ganglia can yield progress on what have been intractable disorders.
Can you please outline the two behavioural paradigms you are researching and your main findings so far?
People with Parkinson’s have a few cardinal symptoms. One of the most pronounced is bradykinesia, which means slowness of movement. Bradykinesia had been studied quite a lot in the clinical literature and in many of the experiments Parkinson’s patients were asked to move a joystick at any speed they want in order to quantify speed of movement.
We really wanted to take that clinical paradigm and get as close as we could in mice, so that we could assess whether bradykinesia would manifest in similar ways in disease models in mice and also to try to understand fundamentally what was going wrong in bradykinesia.
We found that affected mice, just like people, are perfectly capable of making rapid movements in the sense that their motor system is not disrupted fundamentally in any way. In fact, a patient will willingly try to make fast movements, they just won’t be able to make them as frequently. And so, sometimes when you can’t do something the way you want to consciously, we call that an implicit defect in your behaviour, as opposed to an explicit, or deliberative effect.
We found that Parkinson’s disease model mice very closely copy the human behaviour. They show us that they are capable of making fast and large movements. They just have this strong, we suggest implicit, bias against making these relatively enervated movements.
We think that the basal ganglia works like a volume knob on behaviour, albeit implicitly, so you don’t deliberatively control it. We control the speed and amplitude of a lot of our day-to-day behaviours, this is sometimes referred to as the vigour of our movements.
It is actually quite complicated to build a really nice volume knob, for example you can often pay a lot of money for a high quality stereo amplifier! It is a deceptively hard engineering problem, nonetheless, evolution looks to have stumbled upon a solution around 500 million years ago. So we have a fairly good amplifier, but it is one that breaks alarmingly often.
We have also been interested in a number of other behavioural tasks. One of the areas that we’re particularly interested in involves decisions about how long to persist in waiting for something. We like this problem as it seems like the opposite to movement vigor, but still a kind of volume – you can control how persistently you’re willing to wait, doing nothing, thus it is a similar sort of function.
Imagine yourself at a bus stop waiting for the bus to come but it is not that far to walk, and so you have to make the decision about whether you’re going to wait for the bus or walk instead. Intuitively our decision on whether to wait for this bus is based on how variable it has been in the recent past. If we’re used to the bus being late because it’s rush hour or something, we’ll wait a little bit longer before we decide to walk. But, at some point, we make a decision that the bus isn’t coming.
We used the same sort of intuition with mice in our experiments. We would train them to sit and wait for whether a little drop of water was going to come. Then we made the water highly variable and we asked how long were they willing to wait, and whether they were making smart decisions about how long they were willing to wait.
As our intuition goes, you should wait in proportion to the variance of your past experience. If you are at a really unreliable bus stop, you should wait longer, because it is likely the bus could come in the future. Mice do the same thing. We’ve gone on to show that some of the same logic of this volume knob is a good way to think about how long they’re willing to persist and wait. If we think of persistence as a gradient between being impulsive and persistent, volume is the space between impulsivity and persistence.
Did you test whether the mice would wait longer for a better reward?
We would like to do those experiments, but we haven’t done yet. In principle, if you’re making good decisions, they should be based on your desire for the outcome.
This has been studied quite a lot by other groups besides us and, generally speaking, you should be willing to expend more effort, or wait longer, for good things.
How are these mechanisms impaired in Parkinson’s disease? Do Parkinson’s therapies affect the mechanisms?
One of the reasons we’re particularly interested in Parkinson’s disease, from a basic science perspective, is that the primary insult is the degeneration of only one particular class of neuron. To give you a sense of scale, a mouse brain has on order of 75 million neurons: about 10 thousand of those are something called dopamine neurons in the midbrain and if a mouse loses 7 out of 10 of those, they’re profoundly impaired in essentially all voluntary behaviour.
This is remarkable as it is an incredibly small fraction of all the cells in the brain and there are lots of other cell types in the brain, for example in the cortex, where we can lose a much larger number of neurons, and they’ll have little to no behavioural effect after a lesion, or they will rapidly recover function. It is intriguing to us why the system isn’t robust against this kind of loss.
One of the great achievements in neurology in the 20th century was learning how to treat Parkinson’s disease, either through the direct pharmacological replacement of dopamine, or as we began to appreciate throughout the second half of the 20th century, implanting deep-brain stimulators to resolve the downstream consequences of altering the circuit that the dopamine neurons project into.
Why do you describe the computation in basal ganglia as a “canonical” circuit mechanism?
A fundamental aspect of the neocortex is that there is regional specialisation of functions, for example, we know that vision is in the occipital lobe and so forth. However, over the last century, anatomists found that the neocortex has a highly repeating structure. Torsten Wiesel, who was one half of the team Hubel and Wiesel that won the Nobel Prize for their studies of vision in the 50s and 60s, was very fond of this notion that there might be a kind of key function, or computation that was repeated over and over again throughout the neocortex.
Many other people have found this idea very attractive and they’ve generally referred to it as a canonical computation, or a canonical circuit within neocortex, in that you’re just repeating a basic computation over and over again.
Describing the computation in basal ganglia as “canonical” is a homage to the notion that perhaps basal ganglia also has a simple function, something like the amplifier or volume knob, that has common properties or computation applied across its diverse functions.
What are the next steps for your research?
There’s this lovely quote from Sydney Brenner, “Progress in science depends on new techniques, new discoveries and new ideas, probably in that order.”
In terms of our research, over the last several years, we took some new tools, and then a new approach to create some new ideas that we can then test. I think, to some degree, we’re back at a point where we need some new tools to really pursue those ideas further.
It has been challenging to understand how multiple brain circuits work together. Although the mouse brain is small, roughly half an inch by half an inch, that is still fairly big for the sorts of measurements that we need to make. Engineering optical systems and electrodes that can make measurements both at the very fine scale of microns, the scale of individual cells, but also at this larger scale of roughly a centimetre or two, has been really challenging.
We, and many others, are working on figuring out how to make measurements from diverse positions within the brain, such as micron scale measurements, millimetres apart from each other, all simultaneously.
There has been a lot of progress on this, partly through collaborations between HHMI and the Wellcome Trust, developing new electrode arrays, as well as colleagues at Janelia and SWC that have done amazing work developing new optical systems, better fluorescent reporters and so forth. One of our big interests is to use these techniques to understand which components of behaviour are controlled by basal ganglia, and which components of behaviour interact with other circuits in the brain to orchestrate behaviour.
In some ways you might think it is a little deflationary to say that basal ganglia is just the amplifier, or maybe the volume knob. There’s a lot more to your stereo system than just the amplifier or volume knob. One thing that was important to us was to identify some of the things that we don’t think the basal ganglia actually does. One of the profound things in Parkinson’s disease is that all the voluntary behaviour is disrupted, and so we’ve attributed many functions to basal ganglia, essentially as a consequence of this, and I’m claiming that there is a more limited function there. That means that there are other places that need to be contributing, and interacting with basal ganglia. We’re particularly interested in how these multiple areas are orchestrated together.
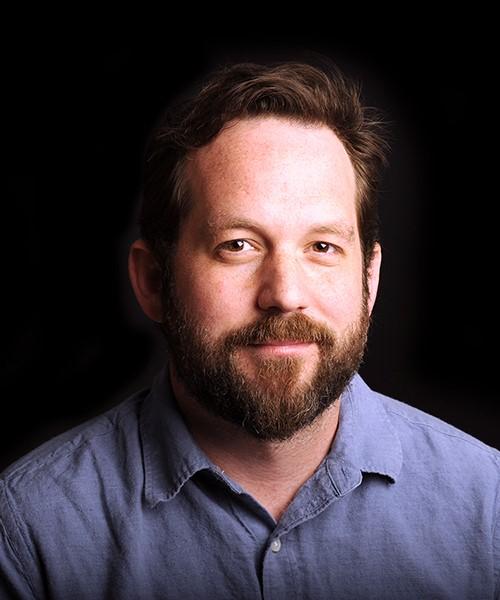
About Dr Josh Dudman
Joshua Dudman received his Ph.D. at Columbia University, where he worked with Dr. Steven Siegelbaum. In collaboration with Dr. Eric Kandel he examined the role of HCN ion channels in regulating the physiology and plasticity of hippocampal and cerebellar circuits. In his Ph.D. studies he also described a non-Hebbian, heterosynaptic form of plasticity in hippocampus – part of an ongoing expansion of our understanding of diverse forms of plasticity mediated by complex dendritic integration of synaptic inputs.
Following graduate school Dr. Dudman joined the Janelia Research Campus of the Howard Hughes Medical Institute first as a Fellow and now as a Group Leader. The Dudman Lab uses a combination of imaging, electrophysiology, behavior and computational modeling to study the role of basal ganglia circuits in controlling purposive behaviors. In addition the lab aims to develop and apply hardware, software, and molecular tools for neuroscience.