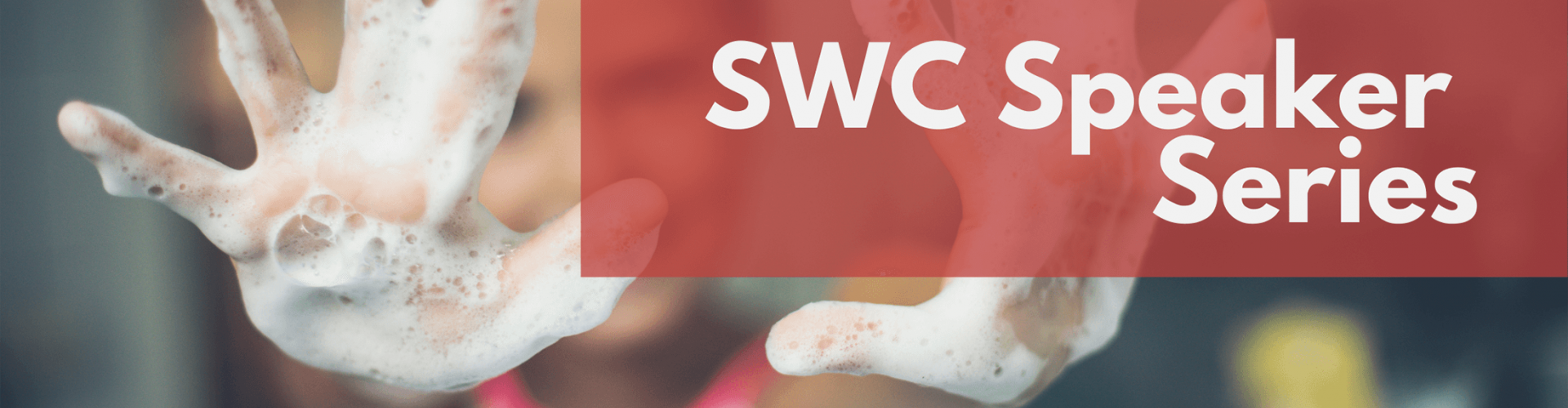
Enabling flexibility in innate behavioural sequences
An interview with Dr Kevin Cury, Columbia University, conducted by April Cashin-Garbutt
Since the start of the pandemic, we’ve been washing our hands more than ever. While the process involves several steps, we simply do it without much thought. But how does our nervous system allow us to carry out such behavioural sequences, while also allowing flexibility?
In a virtual SWC ENSS seminar, Dr Kevin Cury shared his work showing how environmental factors influence flexibility in the behavioural sequence of a fruit fly laying an egg. In this Q&A, he discusses what constitutes a behavioural sequence, what prompted him to study them and his key observations so far.
Could you explain what is a behavioural sequence and how common these sequences are in our everyday lives?
Behavioural sequences are very common in our everyday lives. They involve a recurring pattern of actions. Take handwashing for example: we execute a very familiar and stereotyped sequence where we turn on the water, we scrub our hands with soap, we rinse and then we dry our hands. However, such behavioural sequences must also be flexible. For example, depending on how dirty your hands might be, you may repeat a certain step in the sequence such as scrubbing your hands repetitively.
As I got deeper into this idea, I started to wonder what isn’t a behavioural sequence! It seems like everything could break down in this way at all levels. It is a fundamental part of almost every observable action. There are many actions that people engage in and don’t even realise that there is this structure to them.
Another example is opening a door. In our daily lives we open multiple doors: they might be different types of doors with different handles, and yet they still have an underlying structure. You reach, grab, twist, and open. But flexibility is still required because not every door is the same. There’s a fundamental sequence, but the key is the flexibility behind how and when to transition between components. If you really break down acts as seemingly basic as eating or cooking, they consist of repeating units of behaviour.
What first inspired you to study these behavioural sequences?
I was inspired by the work of Nikolaas Tinbergen and early ethologists to think about flexibility in innate behavioural sequences. Through evolution animals have evolved a broad repertoire of behavioural programmes to satisfy essential goals such as feeding and mating. What’s interesting about these behaviours is that although they’re genetically programmed they exhibit considerable flexibility, which is essential to achieve the goals across varied environmental conditions.
I was interested specifically in fruit fly behaviour and how they lay eggs. Initially I hoped to find a very simple sensorimotor reflex that governed egg-laying. But as I developed methods and analyses, watching the behaviour to really understand what was going on, it became clear that the behaviour had a recurring structure and that laying an egg was not a reflex but a consequence of many layers of built-in behavioural programmes. I would have to appreciate the logic of those programmes to actually understand how a fly lays an egg. The sensory aspect alone wasn’t going to get me there.
Why is it important to understand the neural mechanisms that enable flexibility within the progression of these behavioural sequences?
These kinds of sequences are ubiquitous in life – we engage in them hundreds and thousands of times a day. In every action that we take, there is a fundamental underlying structure. By studying flexibility in the context of flies laying eggs, the hope is to uncover some kind of general principle that might explain the neural basis of behaviour in a broader context.
What experimental techniques did you use to address this question?
The techniques I used evolved throughout the project. In a sense, I’ve done almost everything that you could do! In the early days, there was a lot of basic anatomy, looking at fixed tissue.
After that, there was a behavioural phase, which was a very exciting and creative one for me. I was a bioengineering major as an undergraduate and had gotten just enough of a feel for designing and building things, including a little bit of programming. So when the time came to create environments to observe behaviour, it unleashed a kind of enthusiasm that I didn’t know I had. 3D modelling, 3D printing, laser-cutting, and interfacing all these with cameras and lights were a really fun and rewarding phase. I got to create something, interface it with an animal, then observe the animal actually do things.
And then there was a phase of imaging and physiology. I worked on an in vivo preparation where I recorded activity in a nerve. That was satisfying but also stressful, as it was a very sensitive procedure. I prefer the behavioural route because those experiments are more robust and not as sensitive to the slightest hand motion.
Following that was a phase that interfaced with the behaviour: optogenetic perturbation. Increasingly, I hope to automate some of the steps where I manually triggered optogenetic stimulation. I watched the fly lay eggs and carefully turned the light on and off at precise moments. It was a very intimate experiment in a dark room, watching these flies! I am developing tools for the future that will make some of these steps a little less demanding.
What were your key observations?
When we saw that the behaviour of when a fly lays an egg was variable, we naturally asked why that is. In my work, we demonstrated one major player, an environmental factor, that contributes to this variability – in this case, the firmness of the substrate on which the fly laid an egg. The variability is actually a reflection of the environment. Making that connection was an important part of the work.
But that was just a starting point, because then we asked how the animal actually appreciates these environmental changes, in my case how the fly translates the sensory information about the substrate into a behavioural response, that’s where the other major findings come into play.
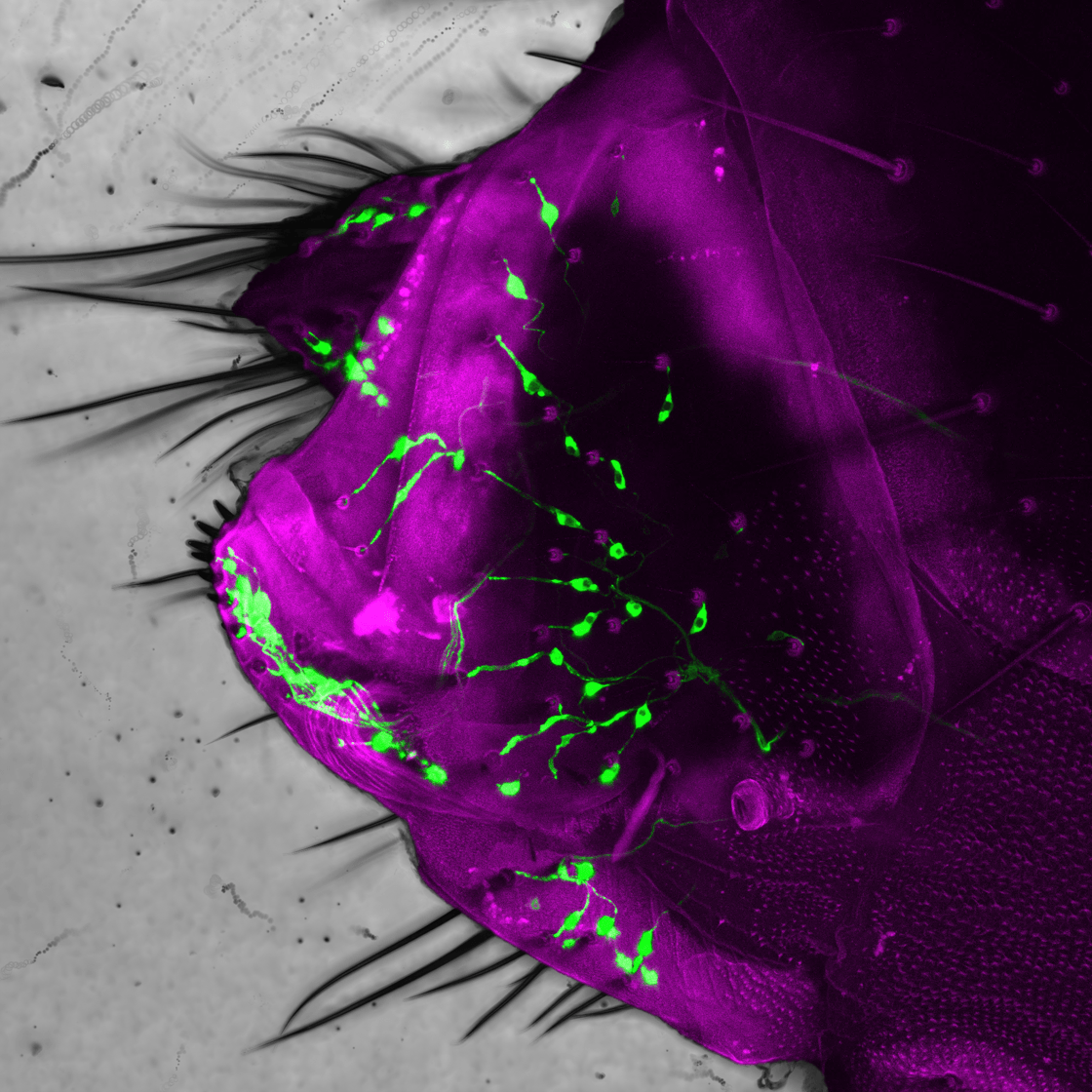
Here we see the posterior abdomen of a female fruit fly (the external cuticle is indicated in magenta). A class of sensory neurons (labelled green) that innervate tactile hairs regulate the progression of the terminal stages of the egg-laying behavioural sequence. Their feedback allows for the sequence to be flexibly adjusted to optimize the placement of the egg across varied environmental conditions.
Sensory neurons that are in very discrete locations of the fly’s body, like the external bristles, or internal egg position sensors, are engaged at distinct moments in the behavioural sequence. And the information that these neurons provide is critical, and in some cases deterministic, of what the animal does.
I think my study provides a unique example of what ethologists call a sign stimulus. But it turns out that the scenario is a little bit more complicated: the stimulus we identified is not a simple trigger for behavioural progression but rather it structures transitions in more than just one way.
What is the next piece of the puzzle your research is going to focus on?
One thing I’m excited to follow up on is answering how the nature or logic of the downstream neural circuit actually integrates sensory information. In particular, I’m interested in how sensory feedback is combined with descending motor commands. I see the two as parts of a puzzle.
The way I think of behavioural decisions enacted in some cases is that the brain sends a high-level command to the spinal cord and that initiates a behavioural action. But the actual execution of that command is a different story. There are examples where a leech is given a command to escape, but depending on the water level, it might crawl or swim. So that introduces this idea that decisions are made sequentially.
I’d like to explore that in more depth: maybe the descending command is like a proposal and the details of what actually happens are worked out below. I’d like to understand how the ultimate consequence of a descending motor command is executed in these lower motor circuits.
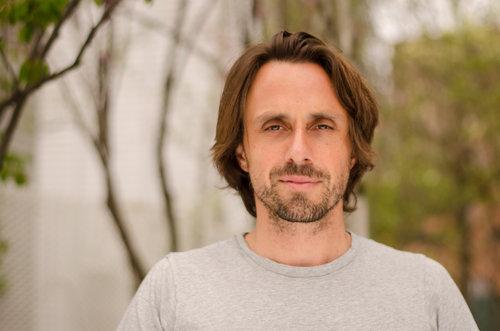
About Dr Kevin Cury
Kevin received a B.S. in Bioengineering from UC Berkeley. Subsequently, he began his neuroscience research career at UCLA as a technician in the lab of Arthur Toga, studying the neural correlates of the BOLD signal. Kevin then pursued and completed his Ph.D. in Naoshige Uchida’s lab at Harvard University. His graduate work examined the relationship between sampling behaviour and neural coding in the olfactory system of behaving rats during odour discrimination.
As a postdoc in the lab of Richard Axel at Columbia University, Kevin is studying egg-laying behaviour in Drosophila as a model system for sensory-motor integration and behavioural flexibility. During this time, Kevin was also a visiting scientist at Janelia Research Campus, collaborating with Barry Dickson to dissect the egg-laying pattern generating circuit.