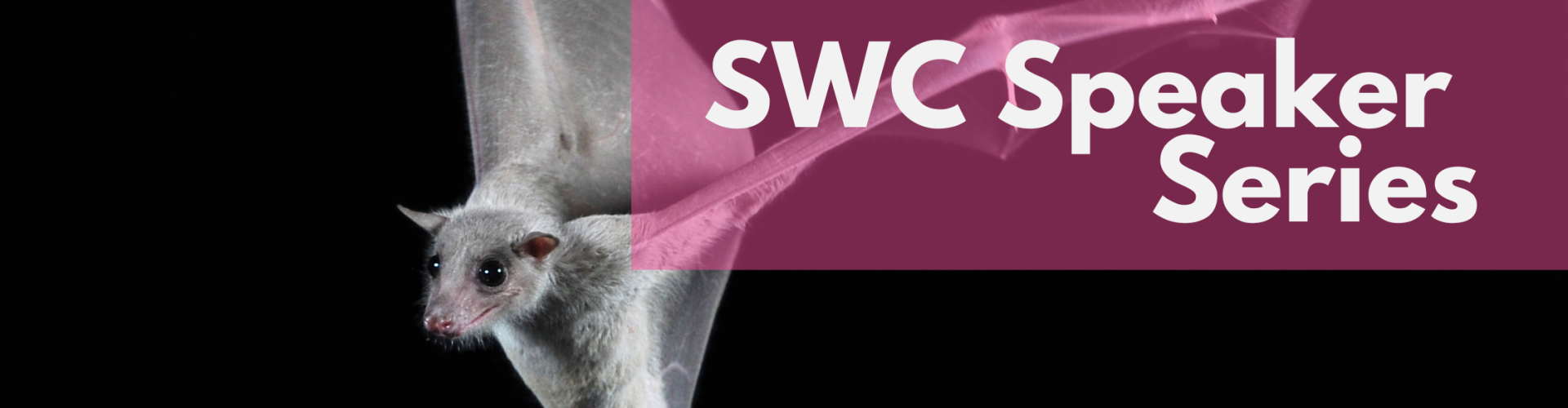
Tunnelling into social codes of the bat hippocampus
An interview with Professor Nachum Ulanovsky, Weizmann Institute of Science, conducted by Hyewon Kim
In the evolving landscape of neuroscience, understanding brain function in naturalistic settings has been challenging. Professor Nachum Ulanovsky sheds light on the recent breakthroughs overcoming these obstacles, focusing on his research with bats. He elaborates on his team’s discovery of how the hippocampus codes social variables and recent experiments on an uninhabited island in Tanzania.
What were the main factors in the early days of neuroscience that hindered experiments in naturalistic conditions?
I think there are two main factors. One is technical and the other one is conceptual. Technically, it has not been easy to record from freely moving animals. Although John O’Keefe pioneered this crucial approach in the 1970s, it was practiced by a minority. For many years, neuroscience predominantly focused on anesthetised or head-fixed animals, or sliced tissue. There was very little data from behaving animals until around 20 years ago.
Technical advancements have made recording from behaving animals easier, especially with wireless technology enabling more naturalistic behaviours. Recent years have seen revolutions in wireless recording methods for both electrophysiology and imaging – and our lab also contributed to this by developing miniature wireless neural-loggers – along with new tools for automatically characterising behaviour using machine learning. Together, these advancements allow for much more naturalistic behaviours and eases their analysis.
Conceptually, there has been a shift towards recognising the importance of studying natural behaviours rather than solely controlled experiments. This recognition has been fuelled by the observation that neuronal responses often differ significantly between natural and controlled conditions. This realisation, coupled with the new wireless technologies and with the development of analytical tools using machine learning, has driven many labs towards studying more naturalistic behaviours in recent years.
Why did you choose to study bats as model organisms?
My PhD focused on auditory electrophysiology, specifically in the auditory cortex of cats, where I discovered memory-related effects. Intrigued by memory, I delved into research on place cells, finding it fascinating but noticing its prevalence in rodent studies.
My inclination to explore alternatives led me to bats due to their unique advantages for studying spatial navigation. Bats' 3D movement, high speed, and sensory capabilities offer insights that are challenging to obtain in rodents. The precise timing of echolocation calls and the changes in echolocation during attention provide valuable data on sensory inputs and on attentional state – which are not easily accessible in other species during free behavior.
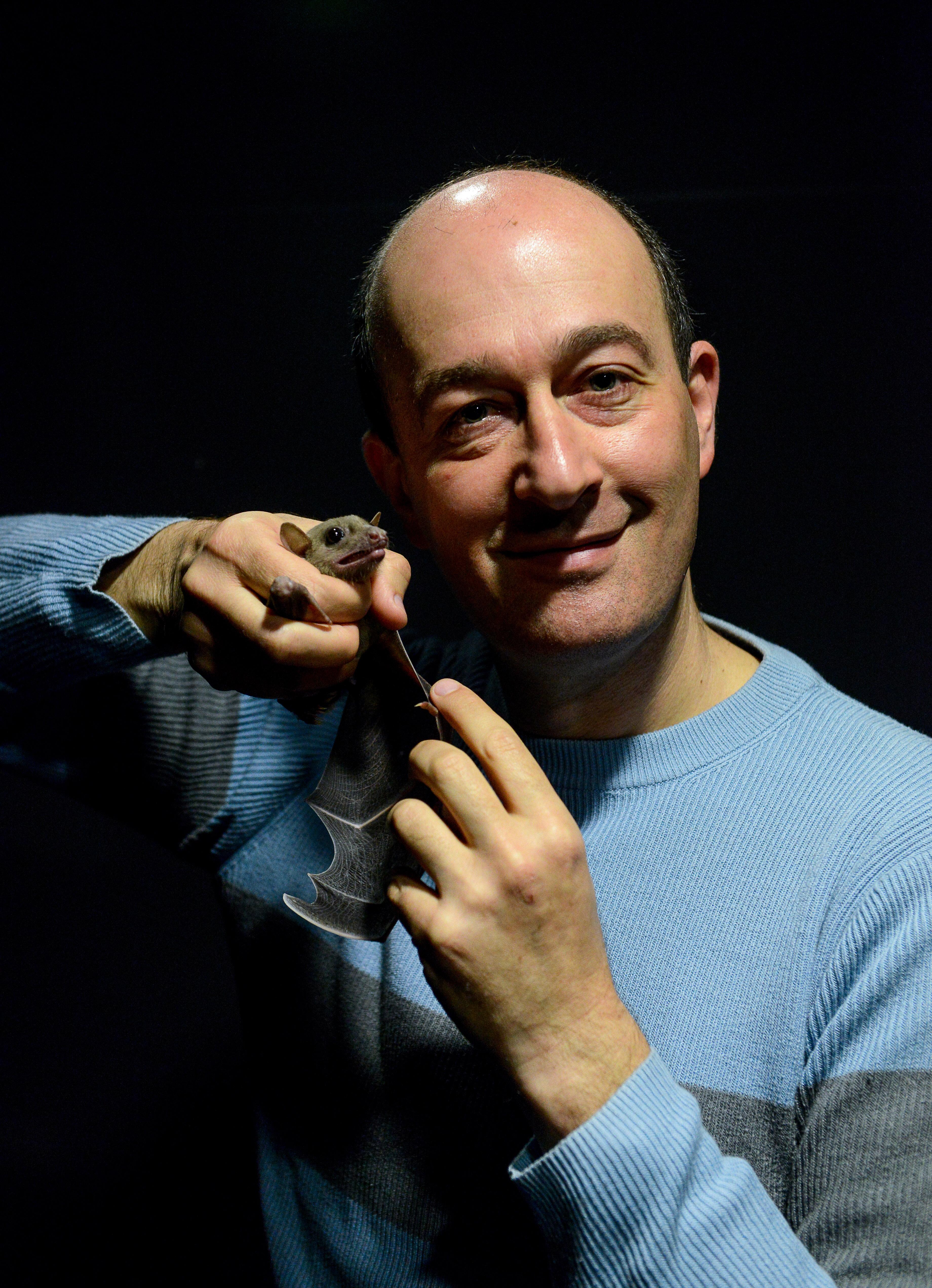
Despite initial scepticism, pursuing bat research proved fruitful, though setting up the lab posed challenges. However, once established, our work garnered significant interest, simplifying publishing and grant acquisition. Overall, while initial hurdles existed, conducting experiments with bats has been quite comparable to rodent studies, with unique advantages that allow novel angles at scientific inquiry.
Could you tell me about how your team developed wireless electrophysiology devices for use in bats?
We collaborate with an engineer named Jacob Vecht at Deuteron Technologies to develop our neural loggers. Jacob handles the electronics while we provide insight into the physiological aspects we aim to study. Together, we've iterated through several logger generations, refining and adding components based on testing and necessity.
Our collaboration is driven by the absence of off-the-shelf solutions tailored to our needs, rather than a desire for technological innovation for its own sake. For instance, we found a suitable off-the-shelf localisation system for our experiments in a long tunnel. However, lightweight loggers meeting our requirements were not readily available, prompting us to develop our own. Over time, we've evolved through four logger generations, continuously improving our tools for experimental research.
In what ways are neural codes for very large environments different from spatial codes in small environments? Why is the code theoretically superior for representing very large spaces?
In our studies involving bats flying in a 200 m tunnel, we made intriguing observations about the behaviour of place cells in large environments. Unlike in smaller environments where place cells typically exhibit activation in a specific location, we noted dramatic differences.
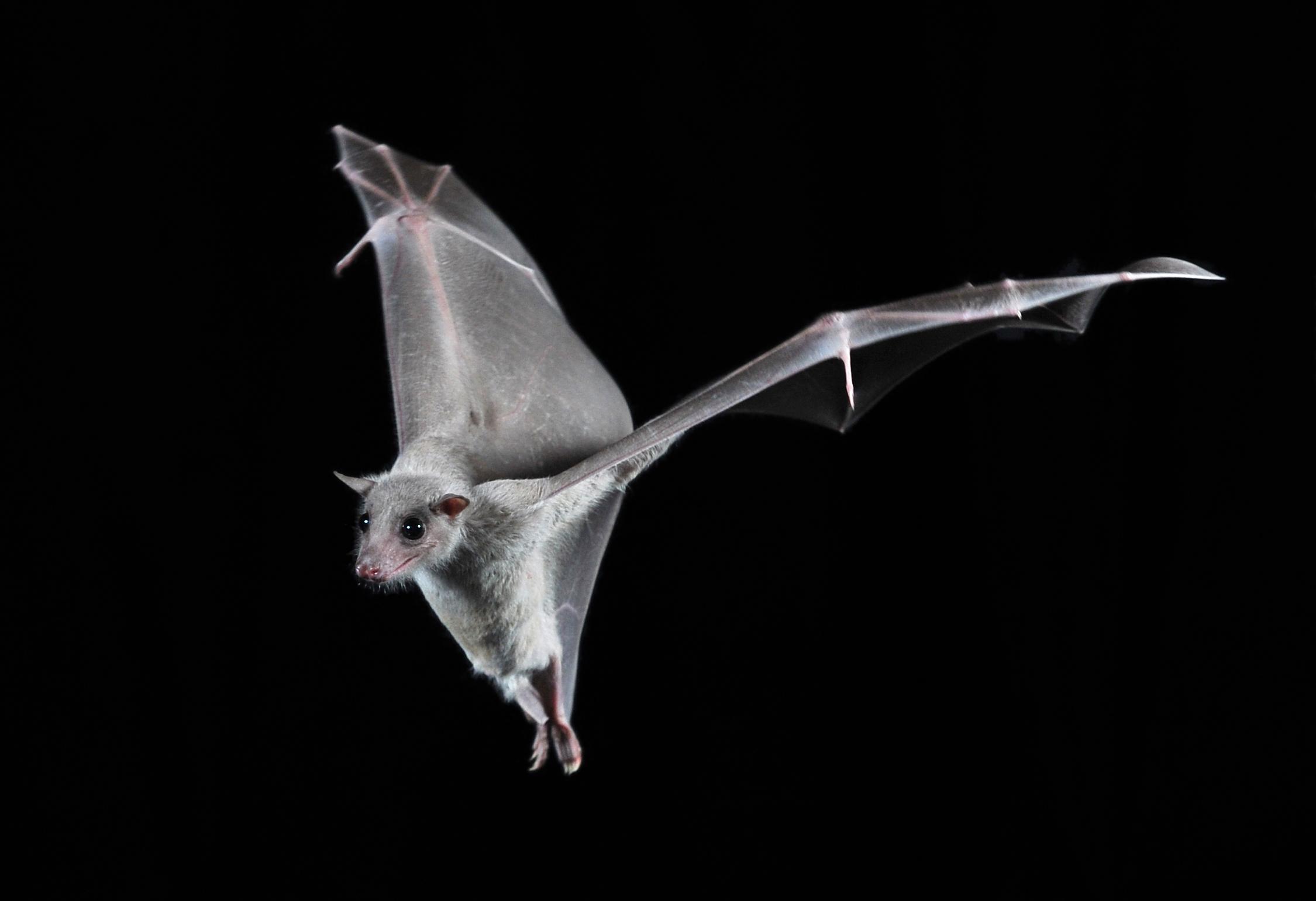
Firstly, we found that in large environments, place fields could be remarkably large, spanning 20 to 30 meters, or more. Secondly, individual neurons could be activated in multiple locations, diverging from the conventional notion of one place field per neuron. Additionally, these place fields varied widely in size within the same neuron, illustrating a multi-scale or multi-resolution coding mechanism. These findings highlighted a new paradigm characterised by scaling up of the field size, multi-field activation, and multi-scale representation – which is distinct from the single, small place field code observed in smaller environments.
Our experimental results and theoretical analysis, published in Science in 2021, demonstrated the superiority of this multi-field, multi-scale code over the classical single-field place code, particularly in decoding accuracy for large environments. This discovery underscores the importance of understanding neural coding mechanisms in diverse environmental contexts.
Were you surprised to find that there is a significant coexistence of spatial and social coding in the hippocampus?
The extent of social signalling we observed in our research was very surprising. While previous studies had suggested a social role for the hippocampus, our findings surpassed our expectations. Not only did we observe representation of both self and others' positions, but also their identity, sex, affiliation, and dominance hierarchy. Neurons even responded selectively to specific social interactions.
This social sensitivity was pervasive, with over 50% of neurons showing significant differences in response to the presence or absence of others during flight. Remarkably, non-place cell neurons exhibited an even stronger social response, highlighting the profound impact of social context on neural activity. The magnitude of these effects exceeded our predictions, emphasising the potency of social dynamics in driving neural responses in the brain – including in the hippocampus.
Importantly, these experiments were conducted in a laboratory setting, albeit in highly social conditions necessitating advanced tracking and analysis methods. While such experiments can be performed in the lab, achieving strong social responses requires setting up a highly social situation – with groups of bats living and interacting 24/7 in the same room – as well as developing experimental methods to track all bats, and sophisticated data analysis techniques. This underscores the critical role of environmental context in shaping neural activity and the ongoing need for methodological advancements in studying social behaviour.
In what way do you interpret your result on a complex neuronal coding of social variables in the hippocampus? How is extreme dynamism in the neural code demonstrated in the bats’ collision-avoidance behaviour?
Our findings on joint neuronal coding of spatial and social variables in the hippocampus suggest that the hippocampus encodes relevant information, whether for memory or other purposes, with greater importance leading to stronger encoding.
For instance, when bats were placed in a highly social environment – living together 24/7 in a group – we observed robust social signals in hippocampal activity. Conversely, during a collision avoidance behaviour study in the 200-meter tunnel, where social identity was irrelevant, neurons exhibited dynamic changes in the neural code without identity coding for the other bat.
This demonstrates that hippocampal neurons represent information that is pertinent to the current behavioural context. In situations where social identity is inconsequential, such as collision avoidance, neurons focus solely on the relevant task of self-localization and avoiding collision. However, in highly social contexts, such as the presence of multiple individuals, neurons exhibit strong social-spatial representation, indicating the importance of social dynamics in shaping hippocampal activity.
The dominance of spatial representation in the hippocampus, which has been found by many labs in both rodents and bats, can be attributed to the ubiquitous nature of spatial behaviour in unrestricted environments, highlighting the relevance-driven nature of hippocampal coding. This emphasises again the importance of considering behavioural context in understanding hippocampal neuronal activity and its role in encoding relevant information.
You talked about some of the reasons why you conducted your recent experiments on an island in Tanzania. Could you share about what led your team to go there and some preliminary results?
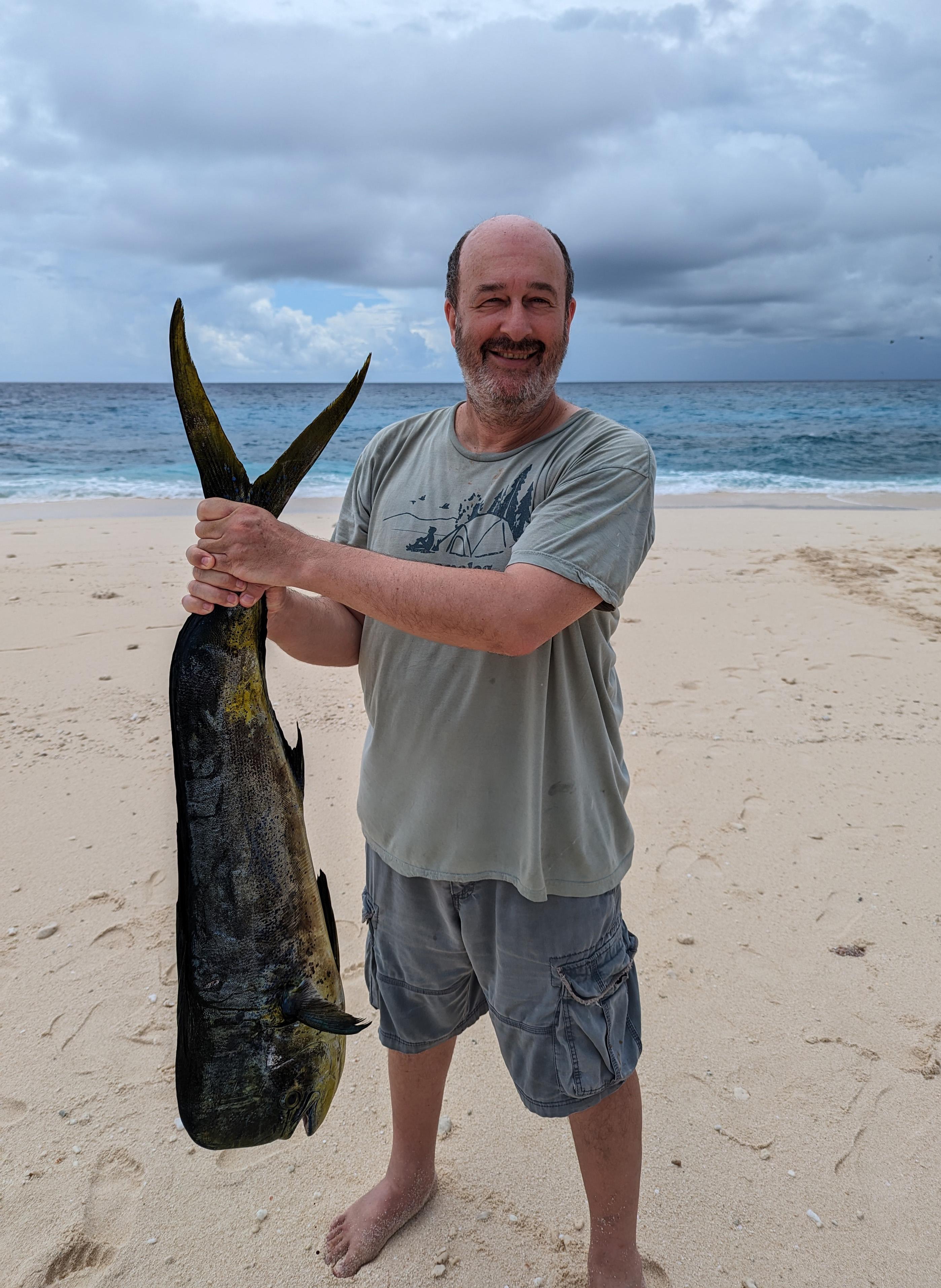
For years, our focus has been on recording from the brain's navigation circuit, the collection of areas where neurons represent spatial information. Given this focus, it was logical to record from animals navigating outdoors, in real-world environments. However, the technical challenges of outdoor recording, such as the difficulty of recapture and the limitation in the number of trials (flight) available per night, both posed significant obstacles.
In 2018, I realised that conducting these experiments on a remote uninhabited island could address both challenges. On such an island, containing and recapturing bats would be much more manageable, and the limited space would ensure multiple flights over the same areas, providing robust spatial coverage.
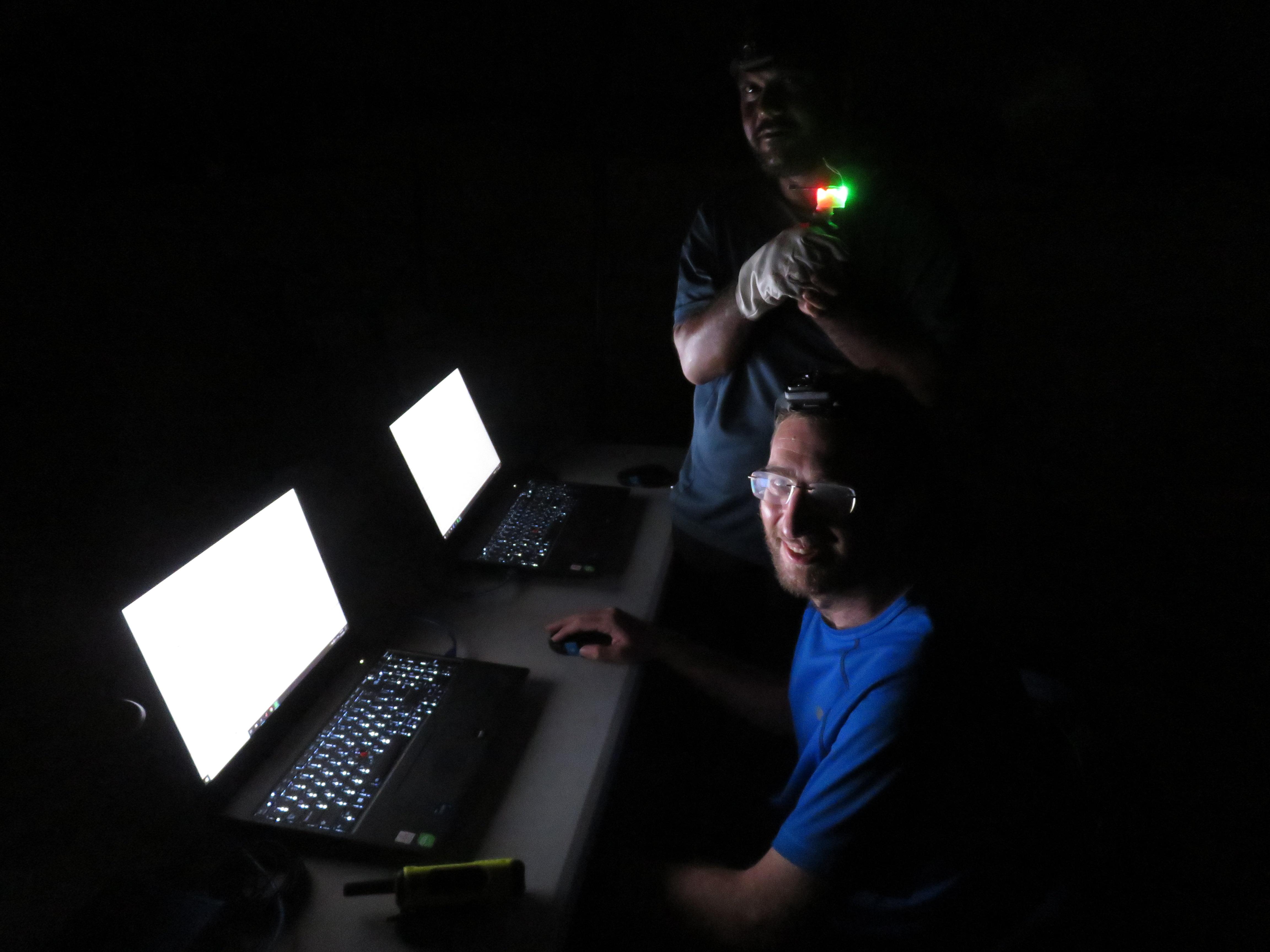
Our recent trips to the island in Tanzania have indeed proven successful, facilitating outdoor recordings with ample spatial data. Our goal now is to investigate whether neurons such as place cells and head direction cells exist and, if so, what their properties are in outdoor settings. While our data analysis is still in its early stages, we are already seeing very interesting results – some of which I showed in my talk at SWC – and we anticipate uncovering numerous insights from these recordings.
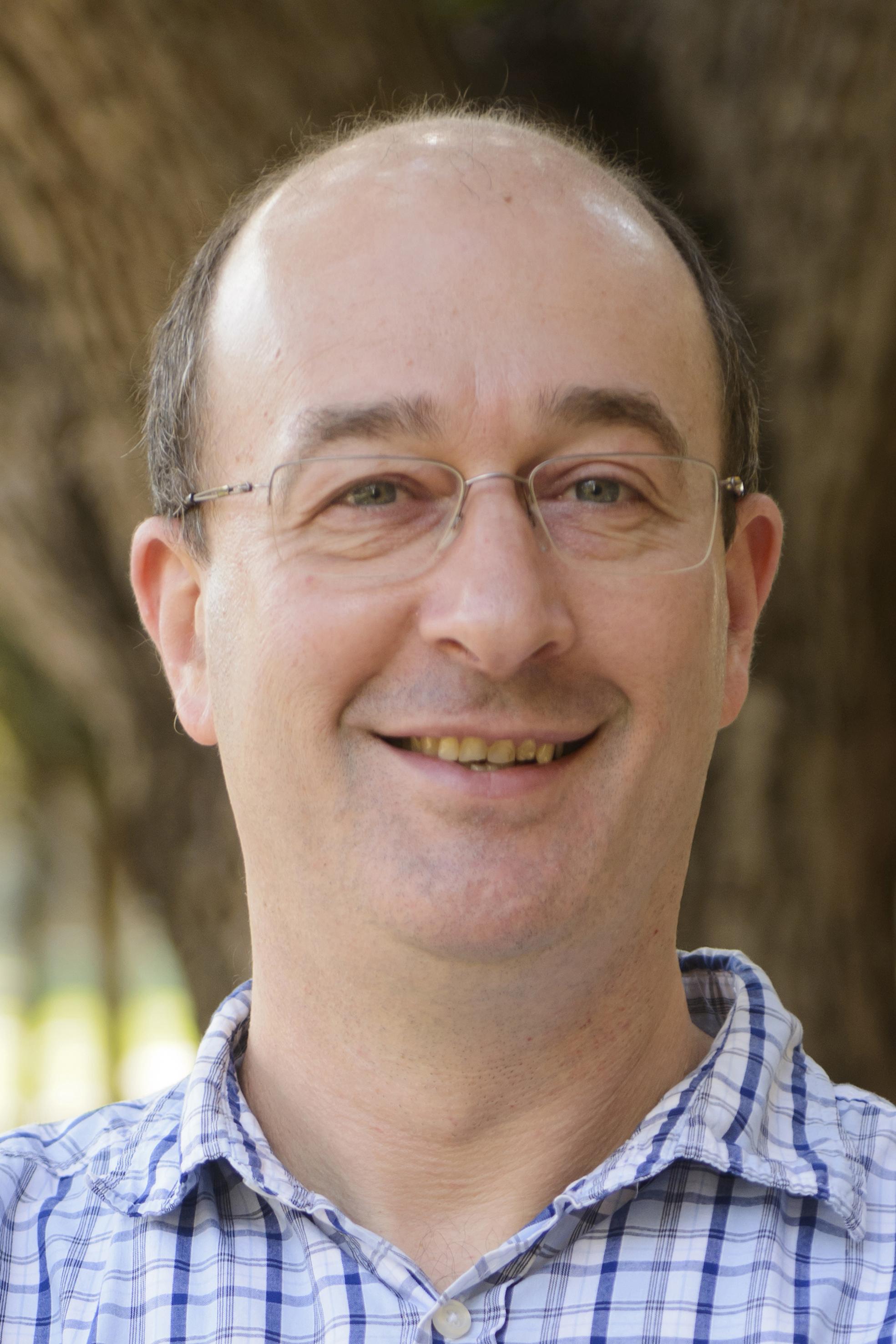
About Professor Ulanovsky
Nachum Ulanovsky is a Professor of Neuroscience and Head of the Center for Learning, Memory & Cognition at the Weizmann Institute of Science. He is an elected International Honorary Member of the American Academy of Arts and Sciences. Nachum studies the neural basis of spatial cognition and social cognition in the mammalian hippocampal formation – using bats as a novel animal model that he pioneered. His group developed wireless-electrophysiology devices, which enabled the discovery of 3D place-cells, 3D head-direction cells and 3D grid cells in flying bats; as well as the discovery of goal-vector cells – which encode navigational goals; and social place-cells – which represent other individuals, in a social context. Nachum performs experiments in bats flying in very large environments, hundreds of meters in size, during navigation and social interactions. He seeks to create a "Natural Neuroscience" approach for studying the neural basis of behaviour – tapping into the animal's natural behaviors in complex, large-scale, naturalistic settings.