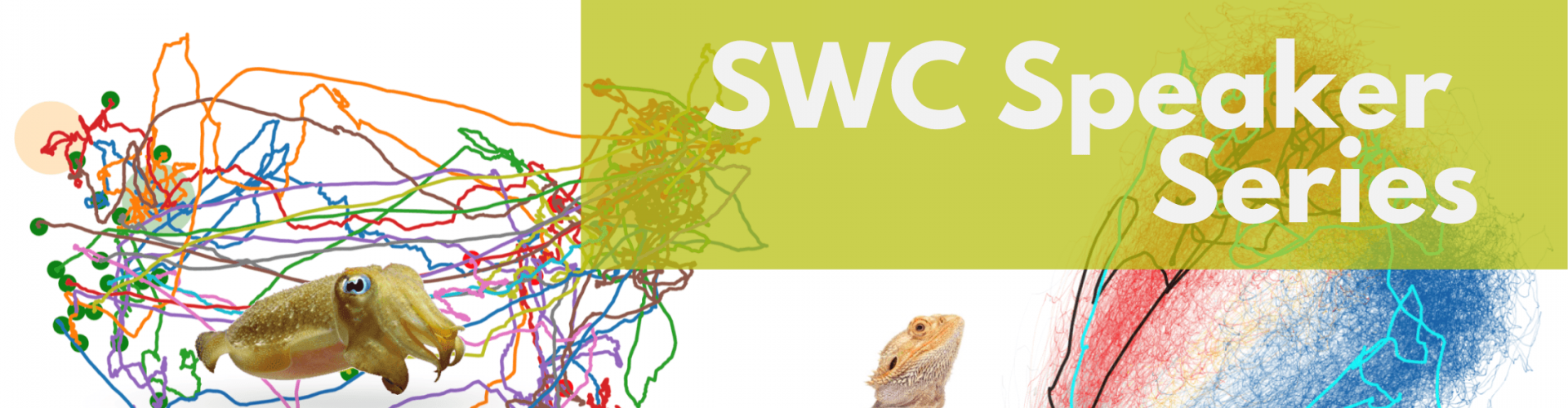
Understanding the dynamic complexity of sleep and visual texture perception
An interview with Prof Gilles Laurent, Director at the Max Planck Institute for Brain Research, conducted by Hyewon Kim
Brain activity during sleep in reptiles and camouflage behaviour in cephalopods: what underlying theme ties these seemingly disparate research areas together? In a recent SWC Seminar, Prof Gilles Laurent shared his work on the neural dynamics in sleep and visual texture perception, using the Australian dragon and cuttlefish respectively as model organisms. The work he presented was accomplished by Lorenz Fenk, Juan Luis Riquelme, Theodosia Woo, Xitong Liang, Dominic Evans, Olivier Fernandez, Sam Reiter and Fritz Kretschmer.
In this Q&A, he elaborates on the routes that his lab took to researching these topics, the specific advantages of using these animals as model organisms, future plans, and more.
What made you become interested in researching sleep and texture perception in the brain? Why are you using the Australian dragon and cuttlefish as model organisms?
We ended up working on sleep by serendipity – we were working on other projects when Mark Shein-Idelson and Janie Ondracek recorded activity during one night and discovered a very regular neural output that piqued our interest. That is how we got into researching sleep.
I was initially not interested in sleep and what we researched had nothing to do with it, but the unusually regular features of sleep in Australian dragon make it a great model system to study sleep and the evolution of sleep mechanisms.
For camouflage and texture perception, that is a very different story. That was a goal to start with and something I had wanted to do for 20 years, but did not have the resources, the time, or other means to do.
So, you could imagine these two research projects as being thematically very different – and of course they are. But there are issues that link them. One is the dynamics aspect - I’m really interested in neural and network dynamics, and both systems exemplify this in different ways. The other is the evolutionary aspect. Having an evolutionary perspective on what the brain does and how it does it interests me and is, I think, important to understand why brains are the way they are.
You’re quite well known for your research of the reptilian brain. Why is the use of reptiles as model organisms well suited for the areas of research you are interested in?
Initially, the choice of working with reptiles was to try and exploit a simpler model of cortical structures. Layered cortices exist only in mammals and reptiles – you don’t see a clear cortical stratified structure in the pallium of amphibians, for example.
The practical aspects of doing electrophysiology and chronic recordings from cortex led us, again partly by serendipity, to Maria Tosches, who came to my lab as an evo-devo person who wanted to probe functional questions. This allowed us to get more deeply into molecular and developmental aspects of evolution. She introduced in the lab single-cell transcriptomics, which enabled us to do quantitative comparisons of cell types between reptilian brains and mammalian brains. This helped us gain a richer understanding of the structure of the reptilian brain from an evolutionary perspective.
For a long time, we had put electrodes in some places in the brain, not exactly knowing what these places were. We referred to classical anatomy papers and decided, ‘We’ll call this area DMT,’ for example – without knowing what it corresponded to in a mammalian brain. You can do this for certain areas, while it is a very uncertain method for others.
The ability to go into such a molecular transcriptomic domain enabled us to find linkages and putative homologies with the mammalian brain. This made things very interesting. We can now look at similarities knowing where we are recording from and compare that with the appropriate target in mouse or other mammalian systems.
How does your research try to understand the dynamics of sleep activity in the reptile brain and the neural basis for texture perception?
We use the types of approaches and methods that people generally use in systems and behavioural neuroscience. That ranges from cellular and molecular to systems approaches, and from chronic recordings to monitoring of behaviour. What is slightly different in our approach to monitoring behaviour is that we monitor motor output in cuttlefish at cellular resolution. Our methods are not terribly different from what others use. It’s an issue that many people now deal with, processing and trying to understand the structure of very high dimensional datasets. Be it recording from 10,000 neurons or 10,000 chromatophores, you are dealing with the same sort of problems and questions in the end.
Because the brain is a dynamical system, it is difficult to understand how the brain works without dealing with the dynamical aspect of its function. So naturally, I am interested in dynamics. Merging the study of neural activity over time with the activity of neural populations is something I have done for over 20 years. It’s not the only approach that one should use, but it’s a level of analysis that I enjoy.
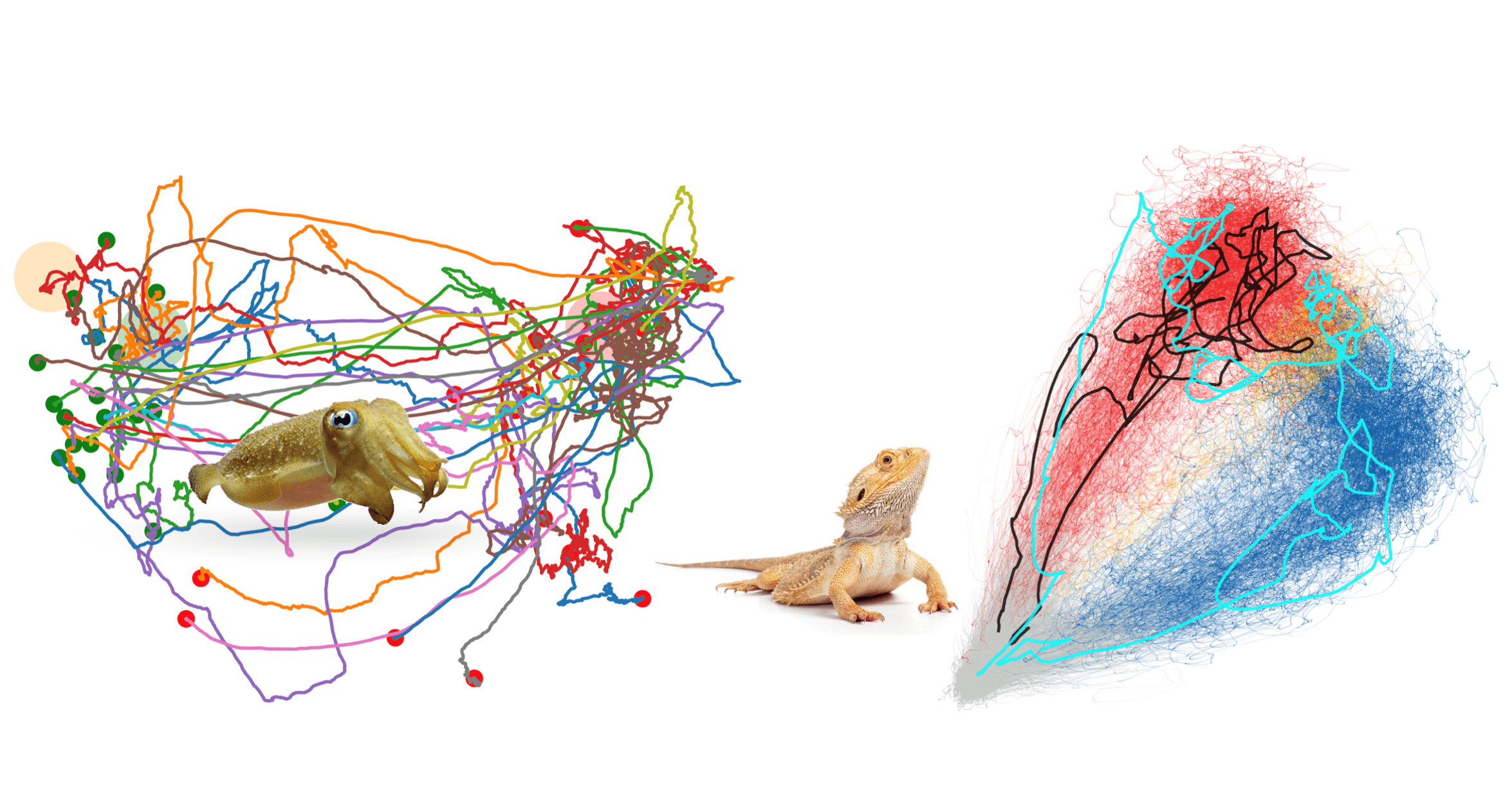
Were you surprised to find a dominance of one half of the brain alternating during a cycle of sleep, or how the texture space contains a tortuous activity?
We were surprised with both. On the sleep aspect, everything we found in these systems when we started working on them was unexpected. First, the existence of REM-like activity in a reptile was new. Second, the phenotype of sleep in this species was also very unusual – very short sleep cycles, similar durations of REM and NREM, the existence of REM itself in a non-avian reptile. All this was new. So was the discovery of the existence of a claustrum in the brain of a reptile. The involvement of the claustrum in slow-wave sleep was also new.
This is the wonderful thing about working with animals that not many people work on. You can be somewhat guaranteed to find stuff that people have not yet found. What is interesting here is that you can relate the finding to neural activity in the outgroup, or mammals, in which most of sleep work has been done.
The new result that I talked about – coordination between the left and right brains – is something that, as far as I know, has not been looked at in any detail in any system. It hasn’t been looked at in sleep, but also in many other aspects of neural processing. In general, people record from one side of the brain and ignore what happens in the other, or the coordination between them. But if you think about it, that’s kind of odd. Representations of hippocampal, visual and auditory processing must all be somewhat matched or coordinated on both sides. How is that done? So, the existence of a competition between the claustra, which we just discovered and is actually transferred from a set of nuclei in the midbrain, has never been described before. It’s fun to discover all these new results.
On the contrary, the cephalopod work builds up on a lot of previous work that has been done over the past decades by a number of labs and in particular the lab of Roger Hanlon at Woods Hole. What is new about what we are doing is that we are doing it in a very quantitative manner, whereby we monitor the states of the animal at high spatial and temporal resolutions so that we can see, not only the state of the output at the chromatophore (cellular) scale, but also the trajectories which they form in pattern space. So, we can figure out how an animal goes from one pattern to another and try to extract information from the path. That was very instructive when examining the variability of these paths through pattern space, their tortuosity and their apparent dependence on sensory feedback. Looking at this at that scale enabled us to find evidence suggesting the importance of some form of feedback during this behaviour. So, the monitoring resolution of the activity of the behaving animal informs us about the putative type of information that the animal uses to get there.
What could this dominance be good for?
This is a big question that you can apply to sleep in general. Sleep is a behaviour that occupies a third of our lives. Yet, we don’t really know what it’s for – we know that we need it, but it’s still a big question. It’s also observed throughout the animal kingdom and presumably must be critical, as it has been naturally selected for. So, that’s a question that all the sleep labs are interested in.
There are hypotheses from a number of datasets in labs that have produced those data. These hypotheses suggest that sleep can be involved in a number of things, such as homeostasis – returning to some kind of healthy equilibrium after high activity during the day or night depending on whether an animal is diurnal or nocturnal; memory consolidation, transfer of memories from one part of the brain to another – for example, from hippocampus to cortex – or erasure of undesirable memories – all these are hypotheses for which there is partial data, some better than others. But there is no definite or real evidence for the purpose of sleep throughout the animal kingdom.
As soon as you start having an evolutionary perspective on sleep, you have to hypothesise that what sleep started as might be quite different from what it has arrived at through 600 million years of Metazoan evolution. All of these different branches might have exploited and used sleep for different purposes. We don’t really know.
These are all open questions. For example, Sam Reiter, a former postdoc who now has his own lab at OIST in Okinawa, studies sleep in cephalopods. Cephalopods do indeed sleep and appear to have two stages of sleep. During one of these two stages, they also form patterns on their skin – during sleep! So, there are lots of potential apparent similarities between sleep in these animals and sleep that we see in mammals and their cousins. Why? We don’t know.
And to return to the detail of your question – the temporal structures that we find that push activity from one side to both sides of the brain in such a way that you get delays in the range of 20 milliseconds or so, by coincidence happen to be a time window that corresponds to a synaptic learning rule that has been discovered in many systems, called spike timing-dependent plasticity (STDP). If pre- precedes post- you typically reenforce the synapse between them; if it’s the other way around, you depress the synapse. So, this kind of temporal structure corresponds to what we find in terms of these delays. That then leads to the hypothesis that maybe such a temporal structure has some role to play in synaptic plasticity – both in reinforcement and in depression. This is only a hypothesis and we have not tested it yet; it is the kind of tentative suggestions that these results lead to.
What implications do your findings have for mammals or even humans?
Regarding sleep, the general process of research is to use a number of different model systems that provide specific advantages. In our case, one of the specific advantages of this system is that the sleep pattern is extremely regular, and one hope is that the kind of circuits that generate these regular patterns might be more easy to study in a model system such as the Australian dragon than it would be in one like the mouse, where REM and NREM cycles alternate far less predictably. So, that is one way in which our system could be useful. But again, it remains to be demonstrated that this apparent competition between the two sides of the brain, suggesting very strong midbrain input to the forebrain during REM sleep, occurs in mammals also. Our results may therefore lead to new research in mammalian systems toward the midbrain to see what happens there.
My approach tends to be not to have too many hypotheses that drive to very specific questions, but instead to let the data take us wherever they take us and only then, form new hypotheses. It is, in most cases, how we proceed – somewhat ill-defined questions in the beginning, then lett things unfold. It’s more fun this way and is a different way of doing science. I admit that it can sometimes be unnerving because you don’t really know where you are going and you need to be a bit lucky.
What are you most excited to work on in the future?
Both research projects that I described today lead to a large series of new questions. Regarding the cuttlefish work, there are a lot of mechanistic questions that have arisen. We now want to look inside the brain and understand how this phenomenon happens. There are questions about texture perception and early visual processing – how an animal can decompose a texture into putative primitives. There are issues of motor control. Are the patterning and strategies that the animal uses made of very specific components and organised in some kind of hierarchy, from the motor unit to larger and larger patterns? Or are they far less organised?
All these are open questions. They are difficult to answer because what we need to do now is to monitor neural activity during behaviour. At the moment, we are working on trying to figure out how to solve that. Dominic Evans, who was a researcher at the SWC with Tiago, is now involved in this project. We are trying to figure out how to monitor neural activity in this system, which is extremely challenging.
Regarding the sleep work, there are a huge number of questions. Some of them have to do with claustral physiology and internal organisation – outputs, projections, convergence. Some of them have to do with mechanistic interactions between the two sides of the brain and midbrain – what are the paths taken that underlie the observed competition between two sides of the brain? Some of them have to do with upstream mechanisms that control the alternation between REM and NREM sleep. This is a big question in sleep research in general. That is one area where I would love to be able to contribute something and is a long-term goal.
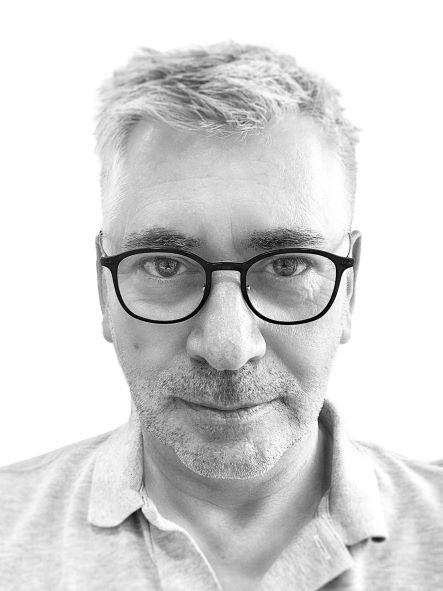
About Prof Gilles Laurent
Gilles Laurent is a Director at the Max Planck Institute for Brain Research in Frankfurt (Germany). Until 2009, he was the Lawrence Hansson Professor of Biology at the California Institute of Technology (Pasadena, CA), whose faculty he joined in 1990. He was a postdoctoral fellow and Locke Research Fellow of the Royal Society at the University of Cambridge from 1985 to 1990. Gilles Laurent’s interests are centred on identifying principles of brain operations. He has worked on olfactory computation in insects, fish and rodents, and on motor control, local circuits, and vision in insects. His present research concerns sleep (in reptiles), vertebrate brain evolution, and texture perception and generation (in cephalopods). A theme that binds these diverse topics is the dynamics of neuronal circuits.