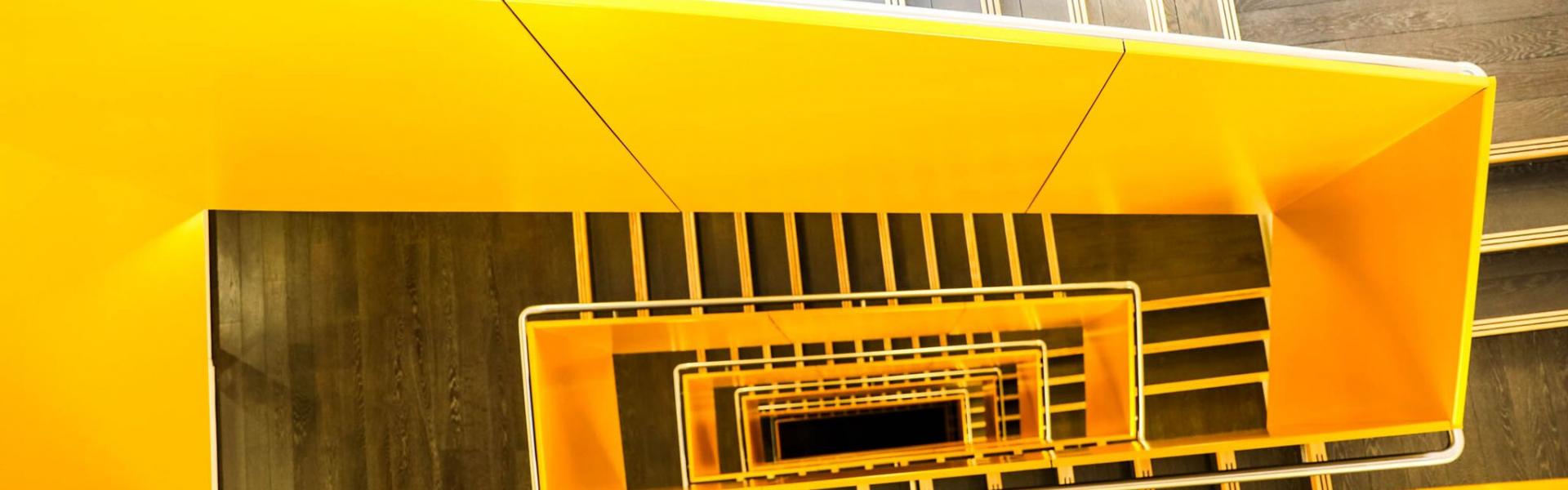
Professor Loren Frank gives scintillating talk on neural substrates of memories and decisions
Howard Hughes Medical Institute Investigator and University of California, San Francisco Professor, Dr. Loren Frank gave a scintillating talk at Sainsbury Wellcome Centre on his research on neural substrates of memories and decisions.
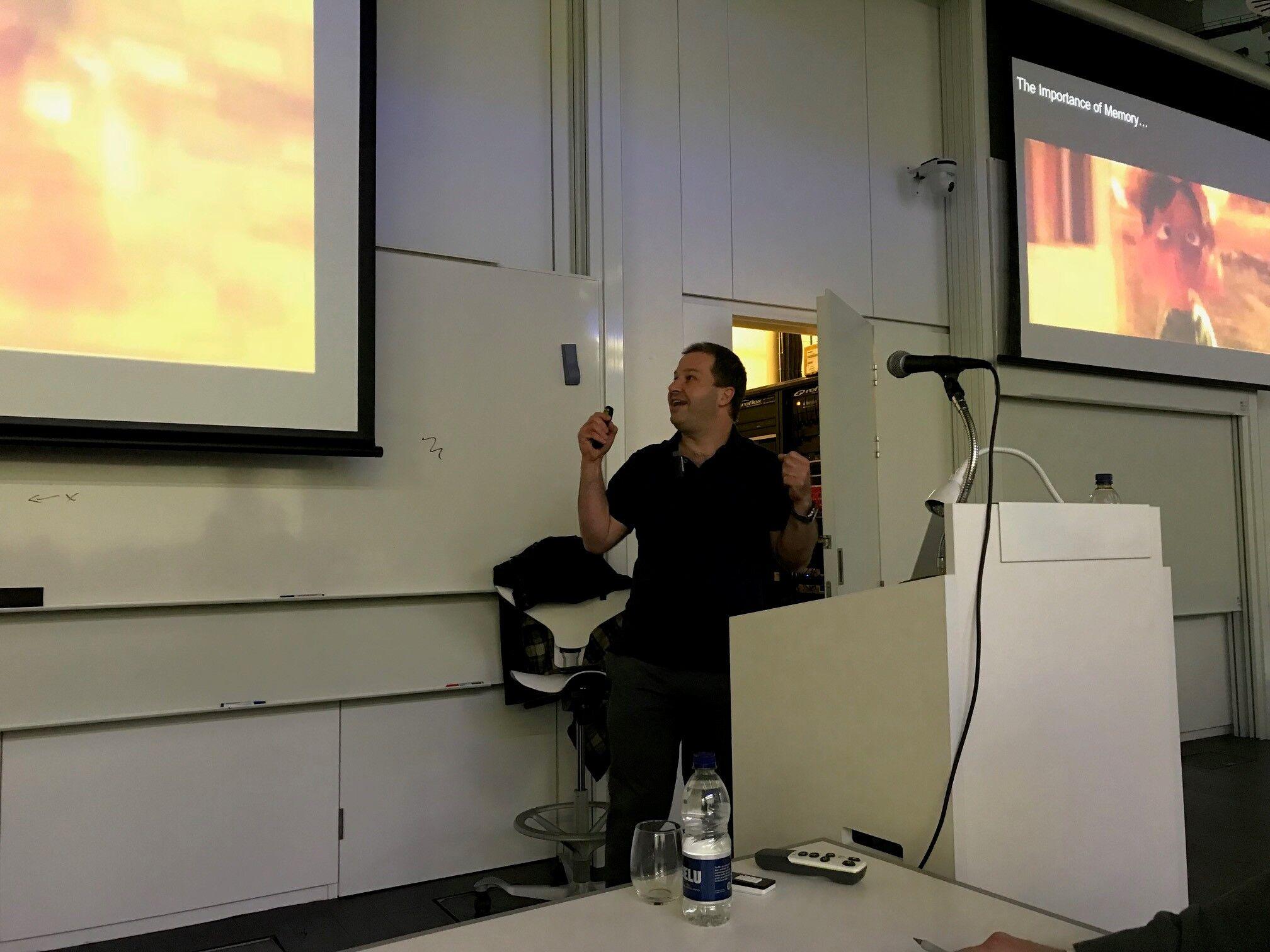
He began by drawing our attention to the familiar feeling of a memory coming back to us in a flash, using an example from the film Ratatouille where a restaurant critic tastes a particular dish and the sensory experience drives an amazing mental time travel back to his childhood.
Professor Frank highlighted how stunning it is that we are able to record our daily lives all the time and that memories that make strong impressions on us can come back to us so vividly and last a lifetime.
To understand how this works, Professor Frank showed a model of information flow underlying memory and highlighted the need for the brain to be able to change quickly in order to store memories. The hippocampus offers this rapid plasticity and is critical for forming memories of everyday life, Professor Frank explained. He also described how the hippocampus is involved in memory retrieval.
Expected properties of memory-related activity
What patterns of activity underlie the ability to retrieve memories? As a starting point, Professor Frank asked us to think about the properties required to achieve memory retrieval. Specifically he highlighted the need for:
- Time-compression – as memories cannot take as long to retrieve as they do to make. For example, if someone asked you what you had for breakfast, you would not take 15 minutes remembering every bite you took, instead you retrieve an extremely time-compressed series of snippets of where you were and what you were doing. Thus Professor Frank demonstrated that memories are only useful if you can take something that happened over a long period and compress it into something useful that you can retrieve to guide your behaviour.
- Representation of future possibilities – memories are useful as they allow you to use past experience to extrapolate more accurately into the future and make predictions about what will happen next.
- Importance for guiding behaviour – memories play a causal role in the guidance of behaviour.
- Activation of coherent representations related to past or potential future experience – this should occur across the brain and not be limited to the hippocampus.
Sharp wave ripples serve as indices of replay events
Professor Loren Frank demonstrated the activity of 46 simultaneously-recorded place cells, which fire in specific regions of space, and showed interruptions in this ongoing location-specific activity in the form of a burst of cells in the hippocampus all firing at once.
These bursts occur during sharp wave ripples (SWRs) and Professor Frank argued that this pattern of activity that could actually subserve memory because you have a lot of cells that are not being place cells anymore, but instead are reactivating in a way that is consistent with the animal’s most recent experience.
To back-up this reasoning, Professor Frank referred to work of his first graduate student many years ago, Mattias Karlsson. The ability to mentally time-travel is crucial for memory, and so Karlsson asked whether SWR events can support this through an experiment. He found that animals can uniquely think about trajectories. This was the first time they found evidence in the hippocampus for mental time-travel, i.e. the reactivation of a pattern of activity associated with a previous experience.
Interestingly the activity of the animal, which took around 30 seconds or so to complete, was replayed in a fifth of a second (200 milliseconds), so SWR events are time-compressed. Professor Frank then demonstrated how this research, along with many other papers, showed that SWR are able to represent future possibilities. He also asked us to think of memory and imagination as two sides of the same coin.
Next Professor Frank outlined a 2012 paper, where they showed that selectively interrupting SWR events during learning, causes a very specific deficit in learning and memory. Also in a subsequent paper they showed the ability to predict, better than chance, whether a subsequent choice would be correct or incorrect based on the intensity in these events. He also mentioned several other papers in the field that suggest links between these SWR events and where the animal is going to go next.
In neuroscience, structures are often studied one at a time, partly for technical reasons but also because each structure is often very complicated. In order to understand coherent representations, Professor Frank described how his lab have been trying to look at the rest of the brain at the same time, so in addition to the hippocampus, they have been recording from early stage auditory cortex, anterior cingulate and prelimbic cortex. They found that the SWR events propagate from the hippocampus to these prefrontal areas, so these are brain-wide events.
Movement-associated and immobility-associated place cells
Next Professor Frank covered the nature of the specificity of these events, i.e. how coordinated they are. He showed a recent result from his postdoc Jai Yu who developed a new foraging task where the animal had to figure out by trial and error which two of the four locations were rewarded and alternate between them for reward, following which the location was switched and so forth.
They found standard place cells that were active during movement – movement-associated place (MAP) cells and instead of excluding times when the animal was stopped, they included them and also found immobility-associated place (IAP) cells that were active and maintained a representation of the animal’s current position when it is stopped. So they saw a fairly continuous representation of space at all times except during SWRs where these things are transiently interrupted.
Interestingly, Professor Frank described how they also found that immobility and mobility representations are activated during different sets of SWRs. Selective activation of IAP cells seems to show the animals are replaying something about an experience that happened at that place as opposed to a sequence of experiences that occur along a trajectory of movement.
Professor Frank described how they found an interplay between ongoing and reactivated representations and when they quantified across all the sharp wave events they saw ~75% only have MAP cells and ~25% have representations only with IAP cells and many fewer than chance have joint activity of both. So movement cells seem to fire together and immobility cells fire together, but movement and immobility cells fire together a lot less.
What does this mean for the rest of the brain? Professor Frank argued that they can use activity during behaviour to predict what the activity might look like during SWRs and which hippocampal and prefrontal cells might be active together. So if movement and immobility representations are broadly distributed that are reactivated largely independently, then immobility SWRs, i.e. SWRs where we see a hippocampal representation of the animal in a place where it was immobile, should activate a population of immobility preferring pre-frontal cortex (PFC) cells, because their content should be coordinated across these sites. Similarly movement SWRs should engage a population of movement-preferring PFC cells. Professor Frank then gave evidence for this prediction of coherent content across brain regions.
Next Professor Frank showed the distribution of cells along two axes – how much they are modulated by immobility SWRs versus mobility SWRs – and demonstrated that there were virtually no cells that had positive modulation of both movement and immobility. Thus showing that in the pre-frontal cortex, far away from the hippocampus, there are populations of cells that respect the same boundaries as in the hippocampus, which suggests that both the content is coherent and that these might be ways that the brain is dividing up experience into different chunks.
To close the loop, Professor Frank then asked what do the pre-frontal cortex neurons do during behaviour? Do they show a truly coherent representation of behaviour? To answer this, Professor Frank showed the average activity of animal coming into a stopping point to get a reward. Specifically, immobility positively modulated prefrontal cells show a spike of activity, just like the IAP cells, right after the animal comes into a stopping point. The movement cells are more active during movement and fall off during immobility. The cells that are inhibited by both, show a pattern of firing that is unlike both, as they come on progressively more the longer the animal is at the immobility location. Professor Frank then showed quantitative comparisons of these three different patterns.
Hippocampal theta sequences
In the next part of his talk, Professor Loren Frank highlighted the problem with the notion that all decisions are guided by SWRs, in that this perspective would be limited to behavioural states where we see SWRs and that only happens when the animal isn’t moving or is moving very slowly. Clearly not being able to think about the future during movement, would cause serious problems. Consequently, Professor Frank asked the related question: are there other forms of this non-local activity that could be useful to inform decision-making processes?
Many people in the field have studied hippocampal theta sequences for a long time and it was originally demonstrated by O’Keefe and Recce at UCL that there is phase precession in the hippocampus.
Activity in the hippocampus can be divided by the phases of the ongoing theta rhythm and thus activity can be decoded. By looking across a large number of trajectories, you can see a sweeping of the mental position starting before the animal’s actual position across to ahead of the animal. This is appealing as a possible way to say something about where the animal is going to be; the problem however, Professor Frank explained, is that we don’t know whether this can represent different possibilities.
To test this, Professor Loren Frank used a standard W maze, where the next correct choice was where the animal wasn’t previously, so the animal had to remember where it was on the previous trial to know where it had to go next. The animals learn that they need to go to the end of the centre arm and then the side opposite the one they visited last time.
Frank then recorded from a number of areas including CA1, CA2 and CA3 and looked at a cross-correlation of the activity of when one place cell would fire relative to the other (both active within movement) both on a long timescale, the behavioural timescale, and a short timescale. In the former, they found that cell 1 fires before cell 2. In the shorter timescale, they found the characteristic data rhythmicity as they fire at specific phases of the theta rhythm. What they actually found was that the black cell (below) fired on every other theta cycle and the red cell is interleaved, e.g. black, red, black, red and so forth.
Then Professor Frank asked how would the brain represent multiple possibilities at once and could this actually represent future possibilities? Through data on virtual trial and error (VTE) behaviours he showed that spiking during these theta sequences actually could represent future possibilities. However, it is very difficult to know whether these are important for anything as in systems neuroscience it is very difficult to do the right causal experiment to find out whether things matter.
Also we don’t know whether these things reactivate coherent representations across the brain. That said, they hope this work will help us understand how the brain in different behavioural states can represent different degrees of potential future thinking or engage that future thinking in the spirit of making these kinds of decisions.
Technology to enable large-scale recordings
In the final part of his talk, Professor Frank discussed the challenges that remain and talked about a technology he has been working on that is complementary to the Neuropixels probes. The particular challenge he focused on was how memory related representations are distributed and the need to measure their activity all at once over the timescales on which memories, and everything else in the brain, are happening.
Professor Frank described how they have been collaborating with Lawrence Livermore National Laboratory to develop a new kind of electrode made from a soft polymer. The probe is a bit wider than the Neuropixels probe, but roughly similar in size. The hope is that this flexible electrode will allow neuroscientists to follow neurons over longer time periods.
The problem with the probe being soft is the attachment in the brain, so a removal stiffener is used to implement the probe. The system can record up to 1,024 electrodes in a freely behaving rat. It contains a stackable series of head stage boards and all of the data comes out on 12 wires on a HDMI cable.
Professor Frank highlighted how they really want to be able to target different regions simultaneously and many of the regions they want to study are not collinear, i.e. they don’t line up, so they want to be able to record from across distributed circuits for long times.
He continued to show that they are able to get recordings for as long as they want to, the example he gave was for 4 months and didn’t degrade over time. They have also taken this out to 10.5 months in one animal and they managed to get single unit recordings for the entire time.
His concluding remarks highlighted the volume of data that this technology and others such as Neuropixels are producing and the need to move into the world of big data to deal with this. One individual soft polymer probe, for example, produces 1.6 TB of continuous data across 10 days, which is about 600,000 spikes. Most importantly, this is out of ~238 simultaneously recorded prefrontal units.
Right now, with the simplest possible matching procedure, they can track ~30% of them over 7 days. This is exciting because there are a lot of questions over what happens during an experience and afterwards and how reactivation evolves over time, that they simply haven’t been able to ask before and now it is actually possible.
Finally, Professor Frank said that although 1,000 channels is great, even with 103 recording channels we are many orders of magnitude off the number of neurons in the brain. They are aiming for 1 more order of magnitude over the next 3 years and have teamed up with Peter Denes, a physicist-engineer at Lawrence Berkeley National Lab who helped design the detectors for cryo-EM.
Denes has built a chip that 1,944 channels of amplifier in 5mm square and now they are working together to reduce to a smaller number of channels so it can be bonded to probes and add digitization. The goal is to have individual devices that might be 528 channels each and a system where up to 16 can be stacked on a single animal and they should be light enough (<1g) so that between 1 and 4 can exist on a mouse.
The hope is that this system will help solve a number of problems, as they can do large-scale recordings that can be distributed and also do long-lasting stable recordings from the same neurons, and ultimately this will enable the asking of new questions.
Contact:
April Cashin-Garbutt
Communications Manager, Sainsbury Wellcome Centre
a.cashin-garbutt@ucl.ac.uk
+44 (0) 20 3108 8028