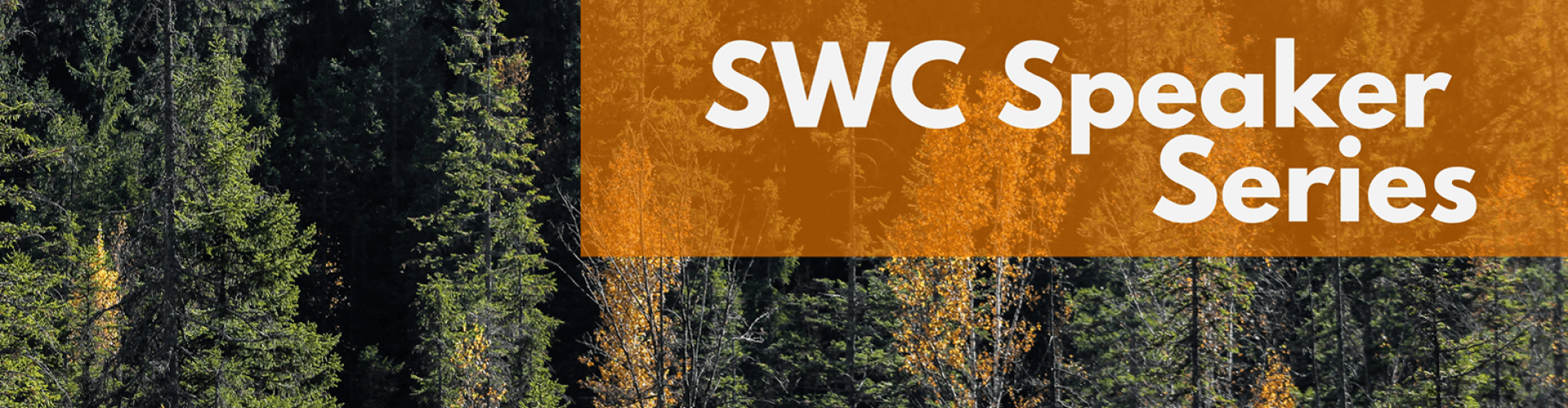
How important is cell type in decision-making?
An interview with Dr Anne Churchland, Professor in Neurobiology at UCLA, conducted by April Cashin-Garbutt
Just like a forest with many types of tree, the brain is made up of multiple different cell types. From the point a neuron is born, it follows a set of instructions that guide its trajectory within the brain. Neuroscientists are beginning to understand how these different types of cell contribute to neural computation. In a recent SWC Virtual Seminar, Dr Anne Churchland shared her work exploring excitatory cell class during decision-making in mice. In this Q&A she highlights how insights from developmental biology led her to appreciate the significance of diversity in excitatory neurons.
Could you start by explaining what you mean by ‘cell class’?
Our brains are made up of around 70 billion neurons and although all of them have in common that they’re brain cells, they’re very different from each other in terms of their shape, where they are in the brain, and – we think possibly – what they do.
It’s easy to look at the brain and say, ‘this neuron’s shape is different from this one,’ or ‘this neuron was born at a different time from this neuron,’ but it’s difficult to connect that cell type to what the neurons do.
Why have previous efforts to understand the neural circuits for decision-making primarily focussed on excitatory neurons and considered them as a single class? Is there a historical or technical context that accounts for this omission?
Until very recently, it wasn’t really possible to know what kind of cell you were recording from. You might have used electrodes to record the electrical activity of the cell, but it wasn’t clear what kind of cell you were measuring. This meant we didn’t have any choice except to think of all those neurons together as a single class.
We now have newer methods that allow us to tell the difference between the types of excitatory cell that we measure and so we can start to connect the properties we’re measuring with the cell types we’ve learned about from the work of others.
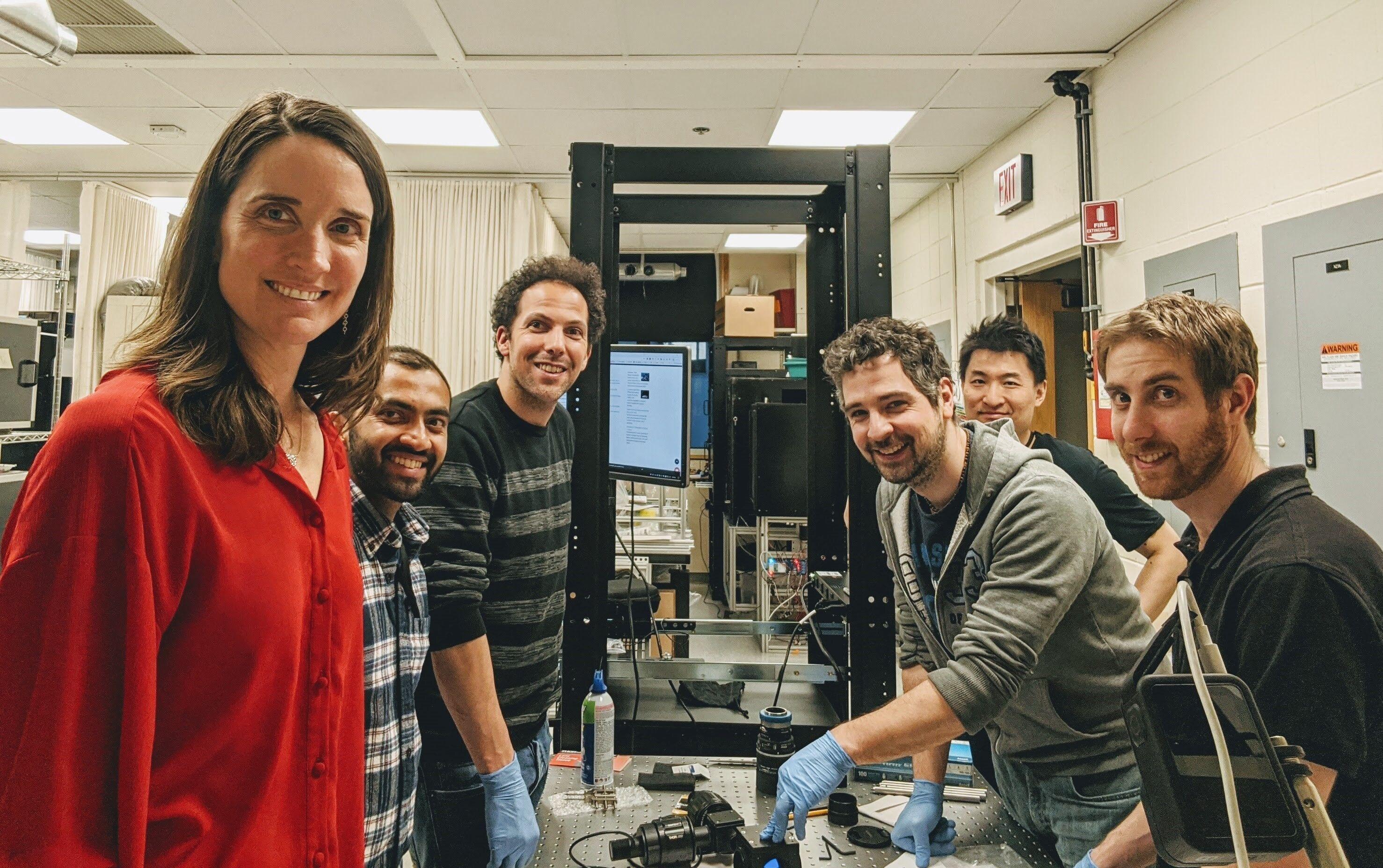
What is currently known about the diversity among excitatory cortical neurons?
A lot is known about how and also why there is diversity. A big part of why excitatory cells are different from each other has to do with what happens in the developing brain.
At the very beginning of life, cells are born in the centre of the brain and then they migrate outwards to where they will live in the adult brain. In my lab, we study the cells that migrate to the outer part of the brain called the cortex. They do this in an interesting way called inside-out patterning: the cells that are born first migrate to the deeper layers and the cells that are born after them go a little bit more superficial, and so on until eventually you have the whole cortex.
Developmental biologists have been studying this process for a long time and have made tremendous progress in recent years thanks in large part to the US Brain Initiative, which allowed scientists to develop really good tools to characterise how these cells are born, where they eventually end up, and what kind of shape they have (called morphology). We now understand a lot about these cell types and what they do in development, but what’s not known as much is what these cells do in the adult brain.
How did you determine whether knowledge of excitatory neuron cell class is relevant to understanding decision-related circuits?
We’re still trying to figure out if it is relevant and it’s not at all a sure thing that it will matter! A naysayer might argue that how cells get to their position in the adult brain isn’t relevant. For example, you might be looking at a pyramidal tract (PT) neuron that expresses a transcription factor called Fezf2. While Fezf2 might have helped the cell get to migrate to the right place, now in the adult brain all the cells are talking to each other, so what difference does the cell type make?
I used to think this way myself. But then, through conversations with brilliant colleagues in very different fields like development biology and also through some work that I did for the Brain Initiative, I really came to appreciate the significance and also the huge body of knowledge about these excitatory neurons and their diversity. And so I started to become enthusiastic about it and to look at a number of papers about what these cells do in the adult brain.
Then I started to think about how widefield imaging could help with this problem as this technique allows us to get a bird’s eye view of the whole dorsal cortex. This seemed to me to be a good tool because we really don’t know what these cell types do, or if cell type even matters. We don’t want to go in with one tiny area in the brain engaged in one particular task. Rather, we want to leave ourselves open to many possible kinds of differences and widefield imaging lets us do that: look at the whole dorsal cortex and see everything that is happening.
We can see it in experts, in novice to expert transitions, and we can use all kinds of different tools – some of which we’ve already developed – to understand how these neurons are contributing to decision-making, understand the spatiotemporal footprint. It seemed like we had a really good pipeline for looking at these cells. So that’s why I teamed up with my former colleague Josh Huang and decided to dig deep into these cell types.
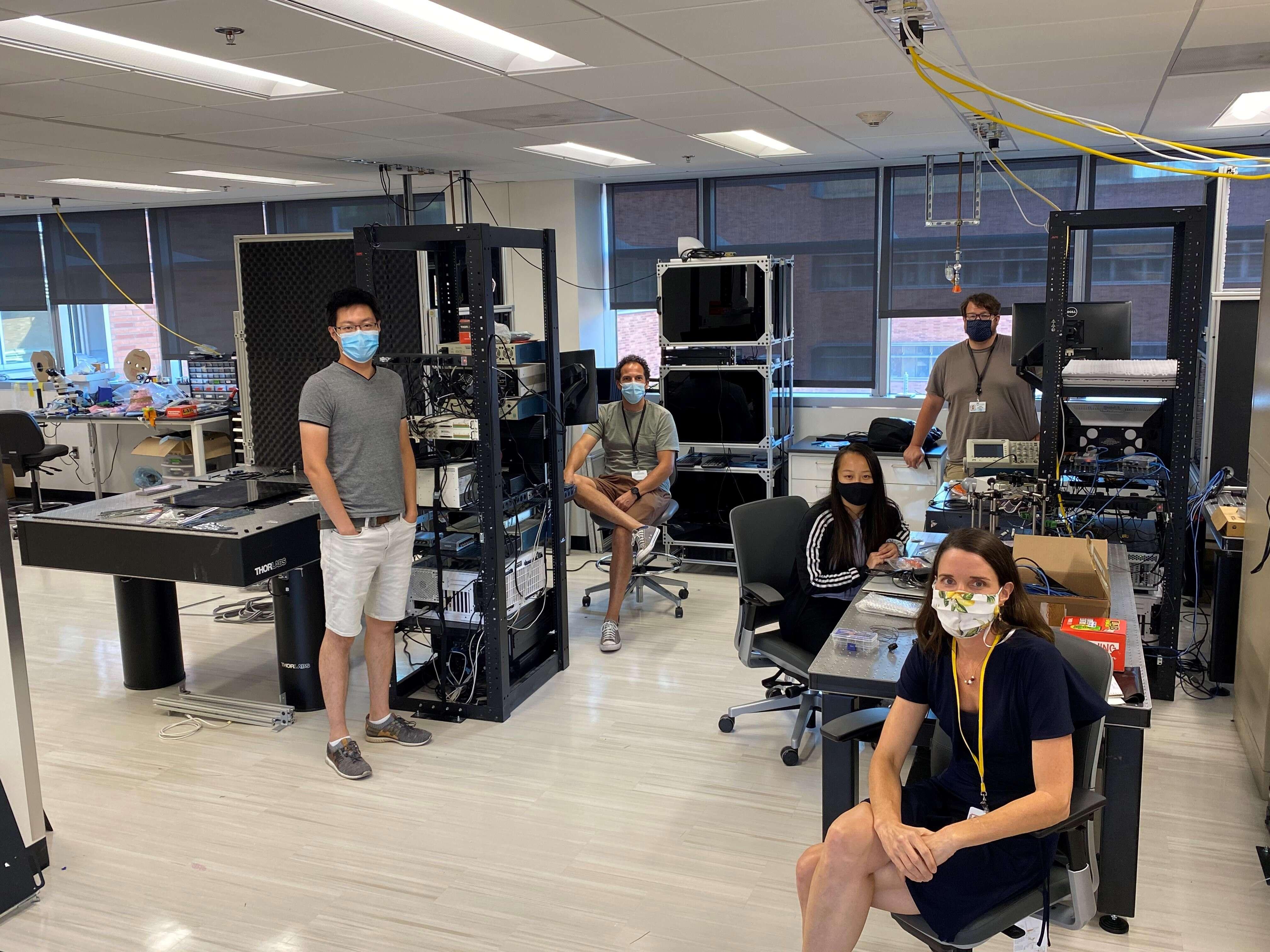
Can excitatory neurons change their class, for example, during learning?
No, they can change their response properties a lot, but their cell is determined by their developmental lineage. What their class means is the set of molecular signals that help them get where they were going back when they were born, so they can’t change their class.
They can change a lot of aspects of their response properties, and they definitely do that, but the way that I’m defining class in this instance: they can’t change. The class is defined by what happened to them during development, so that’s done – it happened.
Based on your observations, how important do you think identifying cell type will be in future experiments?
One thing we know for sure is that these cells are doing different things. And we get a very different picture depending on which cell type we look at, which could mean that this is going to be very important for people to consider when they make their measurements.
But we’re only just learning what those differences are. We think that for example, these PT and intratelencephalic (IT) cells are doing different things around the time of choice (when the animal is making a decision), but we don’t know exactly what computation each cell type is doing, whether one type is doing one computation and the other type is silent, or whether they’re really doing different things. We’re still nailing that down. We know that they’re different, but we don’t totally understand what those differences mean for decision-making yet.
What is the next piece of the puzzle your research is going to focus on?
A few different things! First of all, we want to zoom into the parts of the brain that turned out to be interesting in widefield imaging and measure the responses of single cells. This will allow us to get a close-up view so that we can understand at a much more granular level what’s happening in these areas.
We found that the secondary motor cortex, M2, was a really interesting place to look for differences between PT and IT neurons, but the way we’ll figure out what those differences mean is to zoom in with a technique that allows us to do single cell measurements. Now that we’ve made those measurements, we need to analyse the data and use existing tools and probably develop some new tools to understand what these cells are doing to support the behaviour.
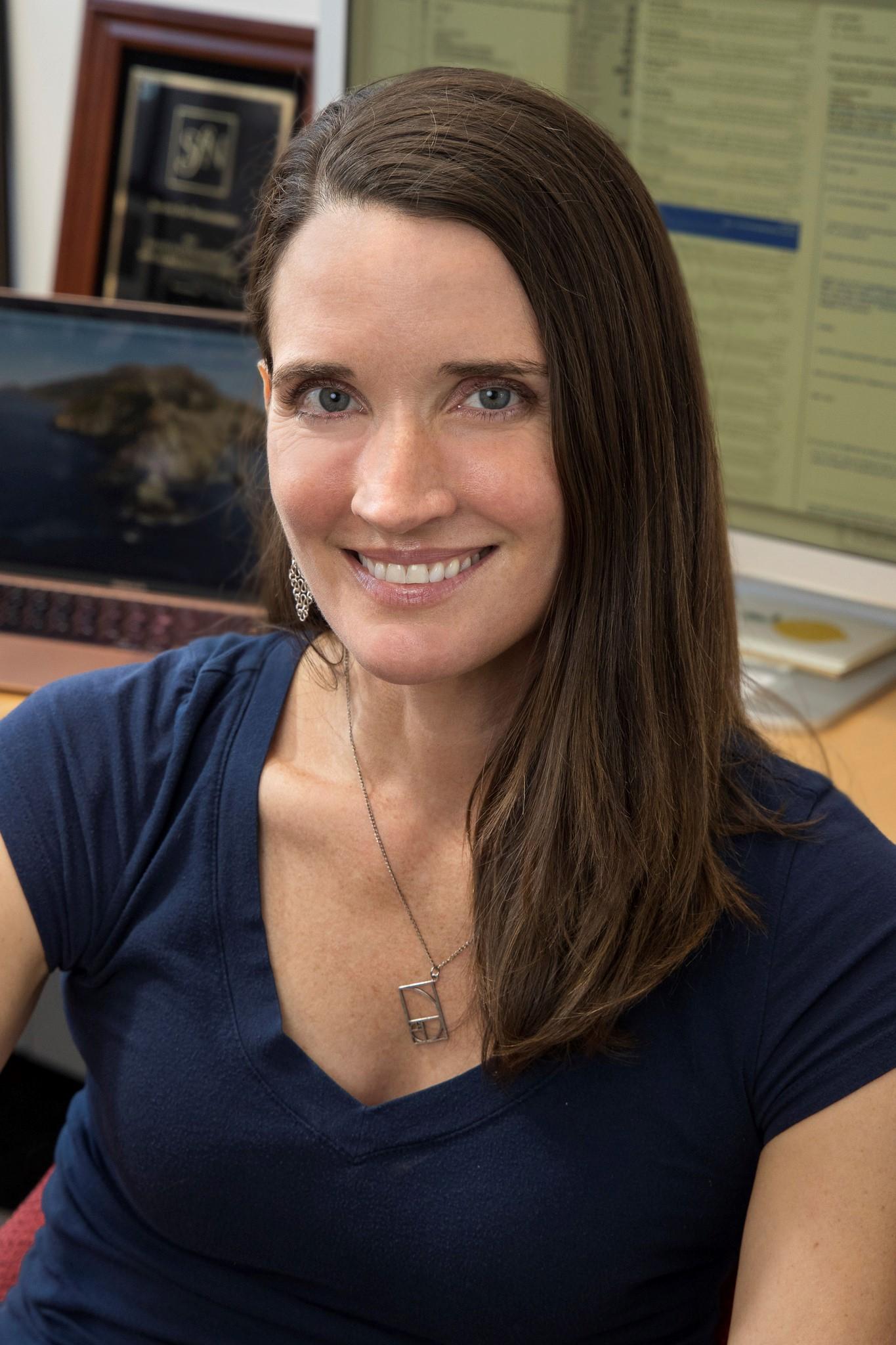
About Dr Anne Churchland
Anne Churchland is a Professor in Neurobiology at the David Geffen School of Medicine at UCLA. Her training included undergraduate studies at Wellesley College, a PhD from UCSF, and postdoctoral training at the University of Washington. She began her independent research career at Cold Spring Harbor in 2010 and remained there until coming to UCLA in 2020. The focus of her laboratory is understanding how auditory and visual stimuli are processed by the brain and used to guide decision-making. She combines experimental work with analysis and theoretical modeling to understand the neural circuits that perform the necessary computations for these decisions. She is also co-founder and executive board member of the International Brain Laboratory, serves on the Advisory Committee to the Director of the National Institutes of Health, and was a member of the BRAIN2.0 Working Group for the BRAIN Initiative.