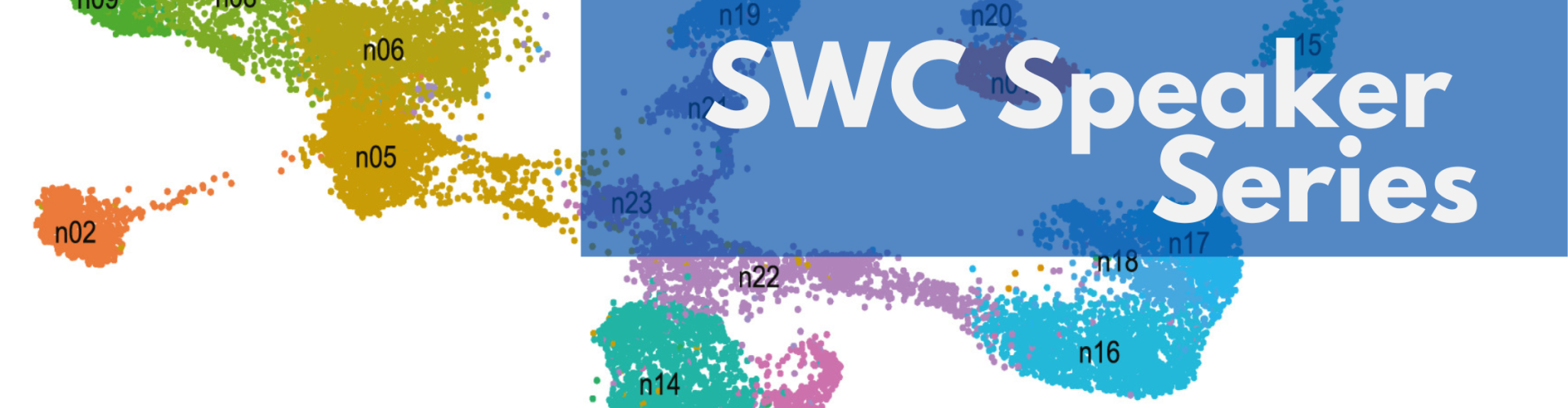
Collaborating towards genetic tools to study visually-guided behaviours
An interview with Dr Jianhua ‘JC’ Cang, University of Virginia, conducted by Hyewon Kim
In the interconnected web of neurons that is the biological brain, some cells excite and others inhibit. An excitatory neuron causes the next neuron to spike, whereas an inhibitory neuron tries to keep the next neuron from spiking. These excitatory and inhibitory neurons are responsible for the computations that our brain performs – allowing us to learn, form models of the world, and plan ahead.
SWC Seminar speaker Dr Jianhua ‘JC’ Cang studies how visual functions, such as our being able to perceive with our eyes, arise from different cell types. In this interview, he shares his team’s findings in the superior colliculus, shedding light on the importance of collaboration and encouraging the visual neuroscience field to embrace the study of tree shrews.
Could you tell us a little bit about the superior colliculus and why you chose to focus on it for your research on vision?
The superior colliculus (SC) is a midbrain structure that is connected to much of the whole nervous system. SC is also a conserved structure, present in fish all the way to primates. It is widely connected through its input and output to many brain areas in all vertebrates. Some of the work that highlights the importance of SC is actually done by many scientists here at SWC. That work, along with those of others in the field, have shown that SC and its connections are important for many visually-guided behaviours. These are the main reasons we decided to focus on SC.
Perhaps more relevant to the mouse, which is the animal model we as well as many of the labs at SWC use, is that most of the projections for visual input from the eyes to the central brain areas actually go to SC. An estimated 90% of all retinal ganglion cells project to SC in mice. That ratio changes in different species, but in mice that makes SC the most important structure for visual processing.
In your talk you mentioned SC is responsible for behaviours like prey capture. How do you take that into account in your experimental setups?
Others in the field have shown SC is important for innate behaviour in mice, including prey capture and escaping from predators or from a looming stimulus. This includes work done by the Branco Lab at SWC.
Most of our own studies were not designed to take those behavioural results into consideration. We’re still primarily focusing on visual processing in the typical laboratory setting to see how visual stimuli evoke responses in SC.
The main finding of ours is that many of SC neurons are direction selective. Whether that direction selectivity is important for prey capture or escape from predators has not been established at all. That would be the next step, to connect those visual stimuli and visual processing we study in the lab to the natural behaviours these animals display.
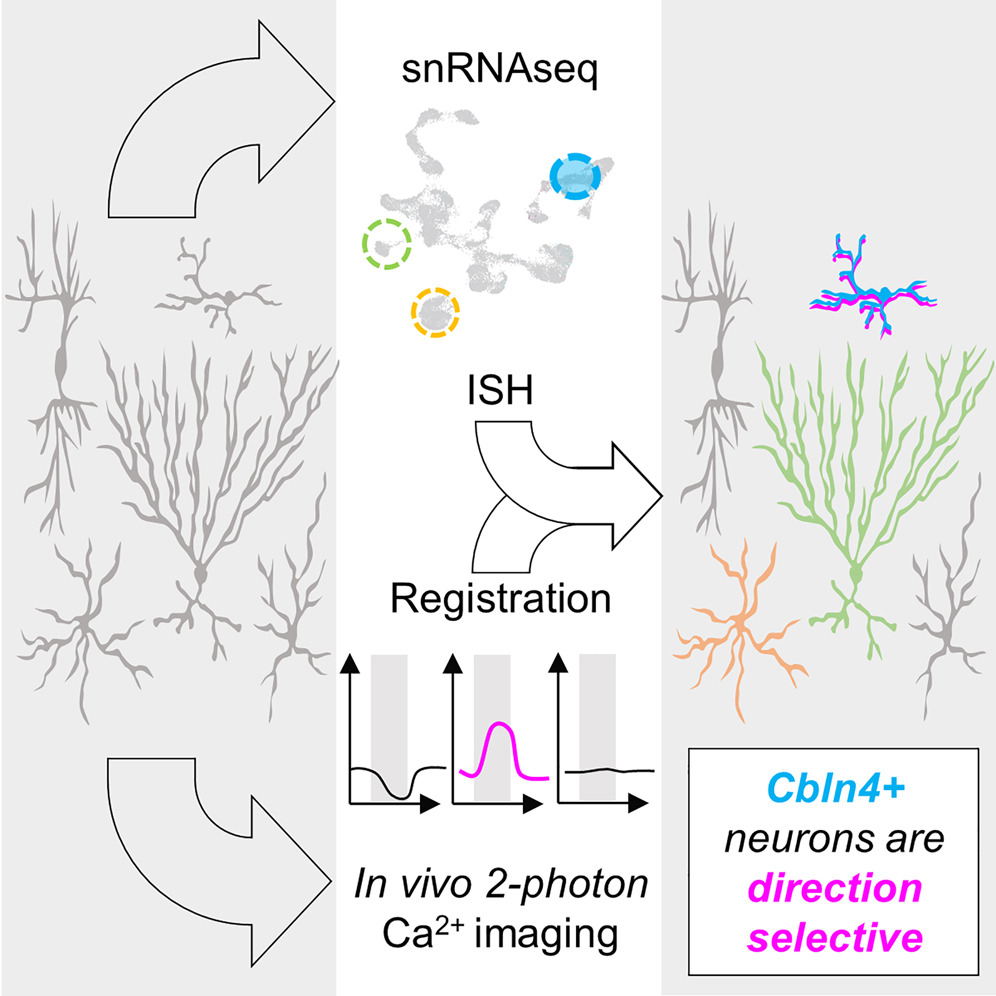
In that regard we are limited in the genetic tools and information on cell types. We did a study to look at cell types in SC, and hope we can find specific cell types involved in direction selectivity computation. We could then take that genetic tool to manipulate direction selectivity and see if these behaviours are affected. We’re not there yet.
How did you get interested in studying cell types in the brain?
There are several reasons. One is a technically-oriented desire to have some genetic access to the functions we study like direction selectivity. For example, if we find a particular cell type for direction selectivity, maybe we can manipulate it. The other is to answer a scientific question: Do we expect certain molecularly defined cell types to display specific visual functions? I think that’s still an open question.
We have some insights and clues now, but it’s not fully resolved yet. There are two examples to illustrate that. First, there are direction selective cells in the retina, which is the input structure to SC. And studies have shown genetic markers for these direction selective cells in the retina. That gave us a genetic logic, if you will, of the basic visual processing in the retina.
But on the other hand, if you go to the cortex where you also see direction or orientation selectivity, as far as we know there are no genetic markers for those cell types and functions. If you compare these two extreme cases, it is an open question whether or not certain direction selective or orientation selective neurons in SC would have a molecular cell type.
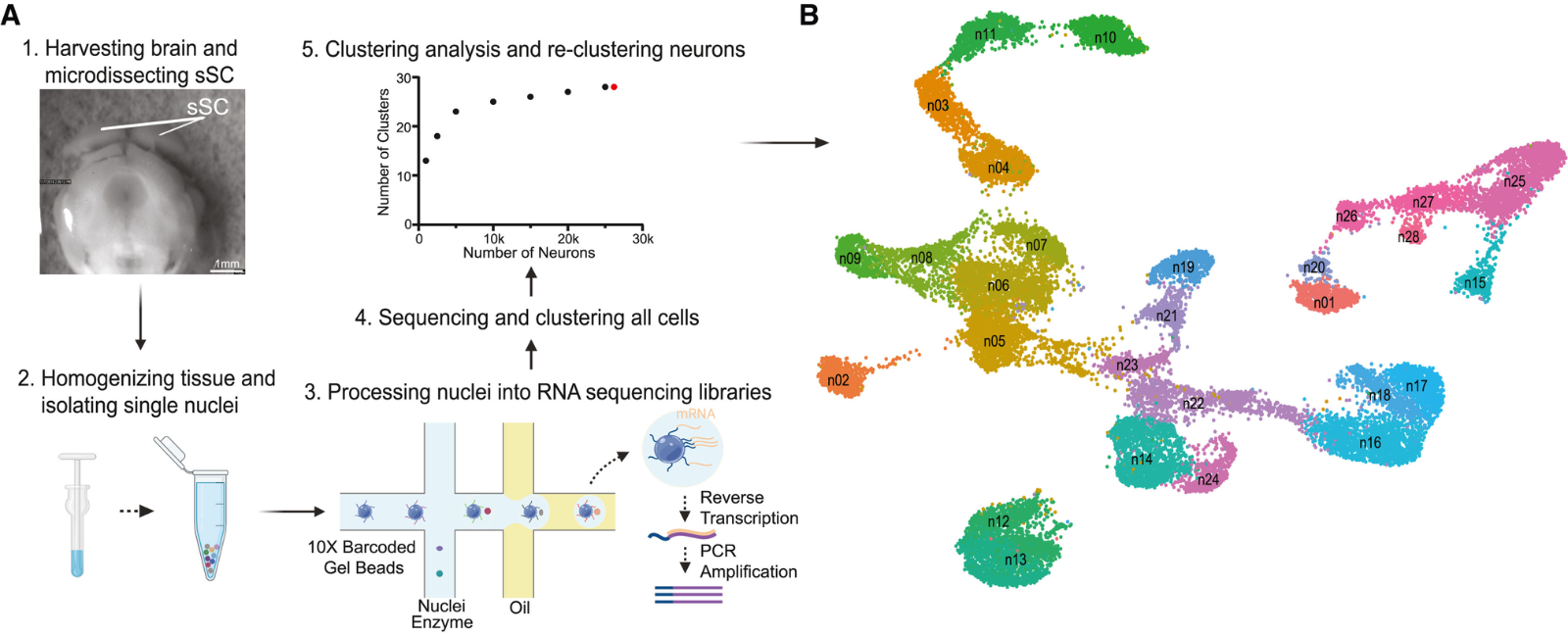
I don’t think there is a straightforward answer or prediction one would have had before this study of ours, which shows how there might be one molecularly defined cell type that is direction selective. I think this finding would advance our understanding of cell types scientifically as well. There were these technical and scientific considerations that got us interested into looking at cell types.
What really made this happen is a collaboration. I, myself, am not a molecular biologist and do not have expertise in that area, although it’s interesting to me and something I wanted to study. But we could not make it happen until we had a collaborator, John Campbell. His lab is expert in single cell sequencing – they look at cell types in different brain structures. We recruited him to the University of Virginia five years ago and the two of us gradually started talking along this direction.
What made the collaboration happen was not only that we were two labs with overlapping interests and complementary expertise, but also that there was an exceptional joint graduate student Yuanming Liu. He was able to get advice from experts in the two labs and make it all happen, with experiments and data analysis, leading to real scientific discovery.
So this type of work takes asking important scientific questions and great collaborations. It also takes students and postdocs driving it forward.
You don’t always hear about the backstories of scientific discoveries like this, what was needed to make it all happen?
I agree. There are many scientific talks where the speaker presents a very clear, polished product. I personally do not like giving those kinds of talks, as I think it’s important to share works in progress – share the struggles we faced and backstories that led to the discoveries in the research. I think it’s a very good lesson for students to hear.
One of the points I made in my SWC Seminar was that we used a particular transgenic mouse line that we all thought was going to work in our experiment. But we found out that it did not, because the cells claimed to be labelled were not. The lesson there was to always check a new transgenic line when you get it. These are things you don’t see in published papers or big talks in conferences. I hope I gave the sense that the work I presented was in progress and still preliminary. I’m hoping to share these learnings with experts in the field and get feedback, as well as share them with students and postdocs doing similar work.
Why was it a big surprise that VIP neurons in the superficial SC are excitatory?
VIP neurons have mostly been studied in cortical areas and they are a well-established inhibitory cell type. There are probably dozens or hundreds of papers on that. This includes a very interesting findings showing that these VIP neurons are important for lots of different functions in neural computation. Given VIP neurons are a subtype of inhibitory neurons in the cortex, we assumed they would also be inhibitory in subcortical structures.
But it turns out they are not! We have solid evidence showing they are actually excitatory, which is a strong contrast to findings in the cortex. We don’t yet know what this means and are still trying to figure it out.
Which candidate marker genes did you focus on in the different layers of SC and why?
All four markers or potential cell types – Vip, Chrnb3, Cbln4+, and Itga7 – have their expression in the superficial lamina of SC, which is the top 50-100 microns. That is where we spend most of our time imaging under the microscope.
This special localisation makes them interesting candidates that we could look at direction selectivity. The superficial laminas are enriched with direction selective neurons. And because we know these four markers are all expressed there, we wanted to understand if some of these four cell types are direction selective. We were able to show that indeed one of these four types, Cbln4+ neurons, are direction selective.
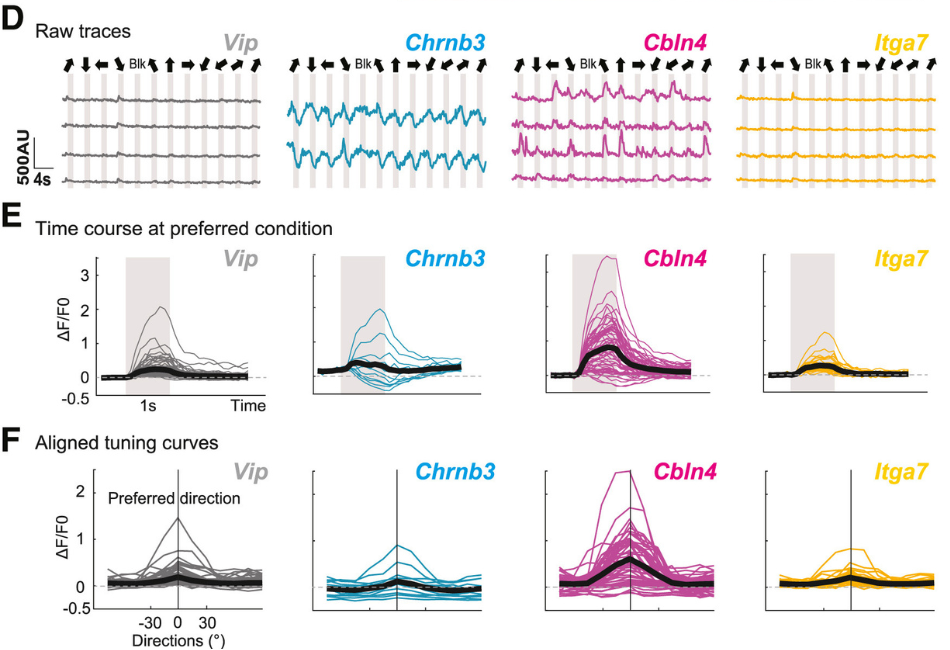
Direction selective neurons in SC include both excitatory and inhibitory neurons. So we have found at least one subtype of direction selective inhibitory neurons, but we have not found any that are excitatory and direction selective. That is what we’re hoping to find next.
What are some big picture implications of this finding?
We have some clues, but no big realisations yet. A conclusion we can make already is that there seems to be a correlation between molecular cell type and visual function. As I said earlier, this was an open question before the study. Now it seems there is a molecular cell type for direction selectivity.
We haven’t found markers for excitatory neurons. The question then becomes whether there is any cell marker or cell type that is excitatory and direction selective. Maybe there isn’t – maybe there is this interesting difference between excitatory and inhibitory neurons in that inhibitory direction selective neurons have a marker and excitatory direction selective neurons do not. That’s certainly possible, though it’s an open question for now.
We’re hoping to rule out or confirm that is the case in the next phase of the study. But if that were the case, that there is this real difference between excitatory direction selective and inhibitory direction selective cells, that could tell us a lot about the computations performed by SC. We also think some of the markers or cell types of direction selectivity are from developmental mechanisms. And if there is a difference between one cell type and the other, that probably means there is a major difference developmentally. I wish I had more to say about this, but it’s still early days.
How do your findings shed light on the development of SC’s role in visual functions in the context of these cell types?
Neural development is one of my favourite topics. I’m interested in looking at how things get wired up during development to display particular response properties.
Our previous research demonstrated that direction selectivity in SC comes from precise connections through inputs from the retina. We showed that direction selective ganglion cells in the retina need to come together in a very precise manner to generate direction selectivity in SC.
What we have now suggested in terms of cell types gives us a way to understand the question of developmental mechanisms behind particular cell types that are direction selective. We could probably ask how these cells respond early in development. Also by looking at their genetic molecular profile early in development, we could see what molecular guidance cues are important for connecting the retina to SC. This gives us a way into answering that question – it gives us a genetic handle on making tools to more precisely get at the answer.
Another part of my lab works on binocular vision. A lot of people in the field have demonstrated that experience is crucial for shaping our binocular vision and depth perception. Children that suffer from disorders that affect their ability to see from both eyes with clear alignment do not develop normal binocular perception, or depth perception. We work on the basic processing and developmental mechanisms of these sorts of properties.
Why did you decide to study tree shrews?
We are interested in the neural basis of vision, but most of the years I’ve run my lab we have been using mice to perform that research. There are good reasons for using mice mostly because of technical reasons – genetic, imaging, and viral tools, as well as behavioural approaches that allow one to study mice in a way not yet available, for example, in primates.
But there are also limitations of using mice to study vision because mice are not particularly visual animals. Laboratory mice we have in our experiments do not have good visual acuity or eyesight, as they are nocturnal and more active at night. Their visual system organisation has some major differences compared to humans or primates in general.
So I think for these reasons mice are a good model for technical reasons but a limited model for scientific reasons.
There has been a collective desire in the field of visual neuroscience to add more animal models to the research. That is why we started working on tree shrews and it turned out tree shrews are much closer to humans. Their visual system is similar to that of primates and they are diurnal, and have higher visual acuity. Their retina is cone dominated, meaning they see colour more richly. For these reasons, their visual system is more advanced compared to mice. This makes tree shrews a very useful model to study for understanding the neural basis of vision.
There are also practical reasons because now, there is a small community of tree shrew research labs. We just had our first in person tree shrew meeting in April 2023, which brought these researchers together. I think this community is growing and we are really excited to bring this initiative to the next level. I’m hoping for more labs to study tree shrews. As a whole field, we need more model systems than just mice.
What could it mean that Cbln2+ superficial SC neurons project to the pulvinar in both the mouse and tree shrew?
This is certainly an interesting finding, but it’s still early days to draw definitive conclusions. Going back to the question of the neural basis of vision, all these years of research have shown there are two main pathways – a primary pathway, from retina to thalamus to cortex, and secondary pathway, from retina to superior colliculus. It’s also known the superior colliculus in turn projects to the thalamus and then goes to the cortex, and that these two pathways could interact.
These Cbln2+ cells could actually be the cells that project to the thalamus. We’ve shown this in mice and we also think it’s true in tree shrews – we need to find out. These cells may be the ones that mediate the interaction between the primary and secondary pathways. It seems these cell types are conserved between mice and tree shrews and this would be a rather interesting finding for scientific as well as technical reasons.
Labs at SWC also study the role of the pulvinar in the thalamus. These may be the cells that mediate the interaction from SC to that nucleus.
What are some future projects you’re excited about?
For this particular project we want to better understand the link between molecular cell type and visual function. Along with direction selectivity we’d like to continue exploring other response properties such as those to visual stimuli more correlated with natural scenes. The larger aim would be to make an even greater link between molecular cell type and visual functions.
The second is to take these steps in the tree shrew, applying the information we have about cell types. Of course, we don’t know much about the visual functions of tree shrew neurons, but one day maybe we can directly compare these properties and cell types identified in the mouse to those in tree shrews. I’d like to know if there are any interesting differences or similarities, both in function and cell type.
And finally, I want to use this information we have and the techniques we developed in this study to answer questions about development.
Do you have any advice for current neuroscience students?
There is a lot of data nowadays. The current phase of neuroscience is big data and there are so many channels for recording spikes and many pixels of images – all a huge dataset. We are in an exciting period of neuroscience research that is unprecedented.
But I do hope the students will take their time to understand how the data was collected and closely examine the quality of the data before they spend all their time analysing to make sense of it.
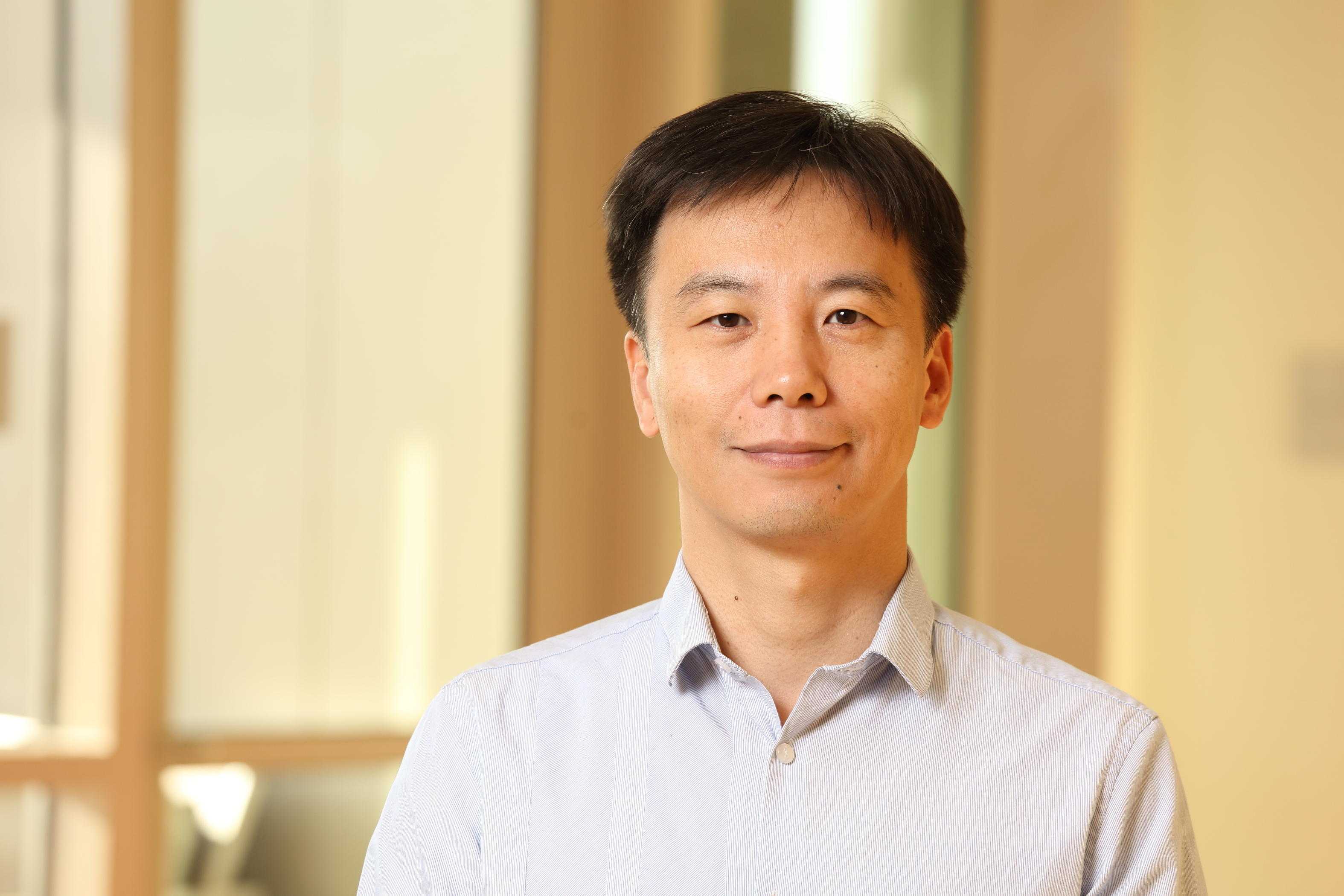
About Jianhua ‘JC’ Cang
Dr Jianhua ‘JC’ Cang received his PhD at the University of Virginia (UVA) in 2001 and conducted postdoctoral research with Michael Stryker at University of California, San Francisco. He started his lab at Northwestern University in 2006, then moved back to UVA in 2017 as a Jefferson Scholars Foundation Professor, with a joint appointment in the Departments of Biology & Psychology. His lab studies the neural basis of vision: how neurons in the brain respond to visual stimuli and lead to visually guided behaviors; what neural circuits give rise to such response properties; and how these circuits are established during development. The lab use mice and tree shrews as models and take an integrative approach that combines in vivo physiology, two-photon imaging, genetics, behavioural, and computational techniques.