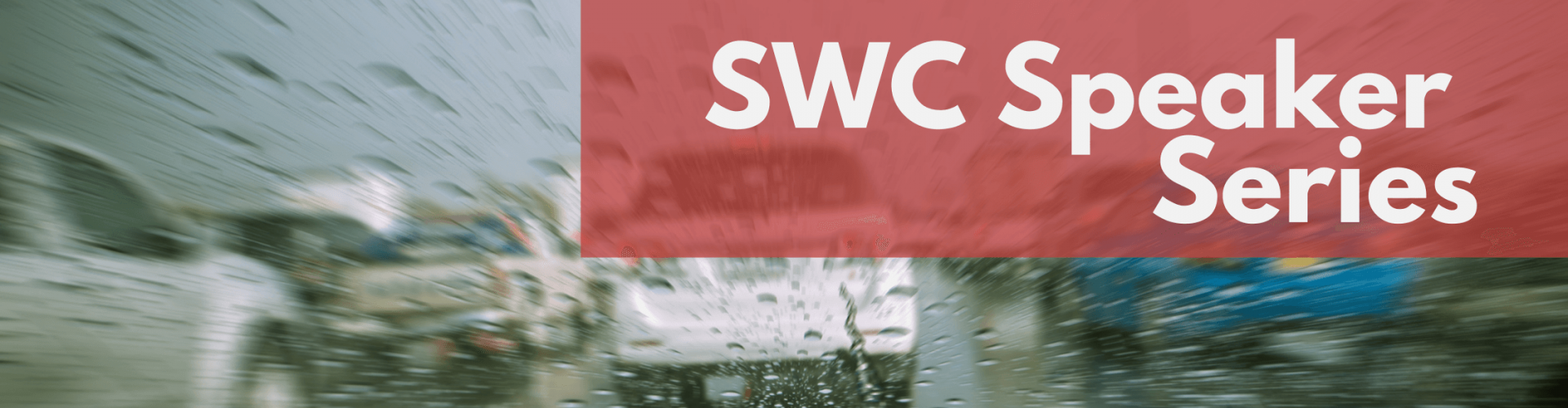
Perceptual decision-making in Parkinson’s
An interview with Dr Michele A Basso, Professor in the Departments of Psychiatry and Biobehavioral Sciences and Neurobiology, UCLA
Driving in the pouring rain is difficult. But if you’ve travelled along the road before, the journey often feels more comfortable than if you were experiencing the route for the first time. This is because your brain can use your past experience to supplement the sensory information you are taking in, thereby allowing you to more easily make decisions and select actions in conditions of sensory uncertainty. But how does the brain actually achieve this combination of past experience and sensory information? And what can we learn about the changes to this process in people with Parkinson’s? Dr Michele Basso recently gave a SWC Virtual Seminar where she outlined her research on these areas, and in the following Q&A she explains more.
How do you define perceptual decisions and what does the brain do when sensory information is unreliable?
The way we define perceptual decision-making is the ability of the brain to acquire information from the senses – for example, how we take in information visually and use it to inform our choices of action. We used to call this sensorimotor integration: how the brain takes sensory information, integrates it, and produces a choice of action based on that sensory information. When you see a fly zipping around, how are you able to swat it? The brain must track the object in three-dimensional space, calculate the motion, perhaps make predictions about where the fly will land next and then turn that information into a pattern of muscle activations to move your joints to take action.
But how do we make decisions and select actions in conditions of sensory uncertainty? You can think about this in everyday life for example when you are driving a car. When the sun is shining and you have good visibility, you use all that sensory information. But what happens when you’re doing the same thing but it’s pouring down rain and there’s lots of fog? Your visibility is significantly impaired, so you’re going to modify your behaviour accordingly by slowing down. But if it’s a route that you’ve driven numerous times, you have some internal representation of where the stop signs are, of how the traffic usually flows in the street, so your brain is able to use and incorporate that memory information to help guide your driving behaviour.
In your decision-making process, you will give more credit to your past experience information more strongly than to the sensory information. And it makes sense that you would do that because the sensory information is unreliable. If you know the road very well, you’re going to rely on that knowledge more heavily than on the sensory information. There’s lots of evidence that this occurs in people, monkeys, rats and mice: they all weigh their prior memory experience more heavily, particularly in conditions of sensory uncertainty.
How the brain actually achieves this is the 64 million dollar question! We think that the basal ganglia and superior colliculus are playing key roles, at least in integrating information. The information itself might be saved somewhere else in the brain, but the basal ganglia and superior colliculus are thought to play a key role in how sensory evidence is weighted and in determining the action outcomes based on the integration of information.
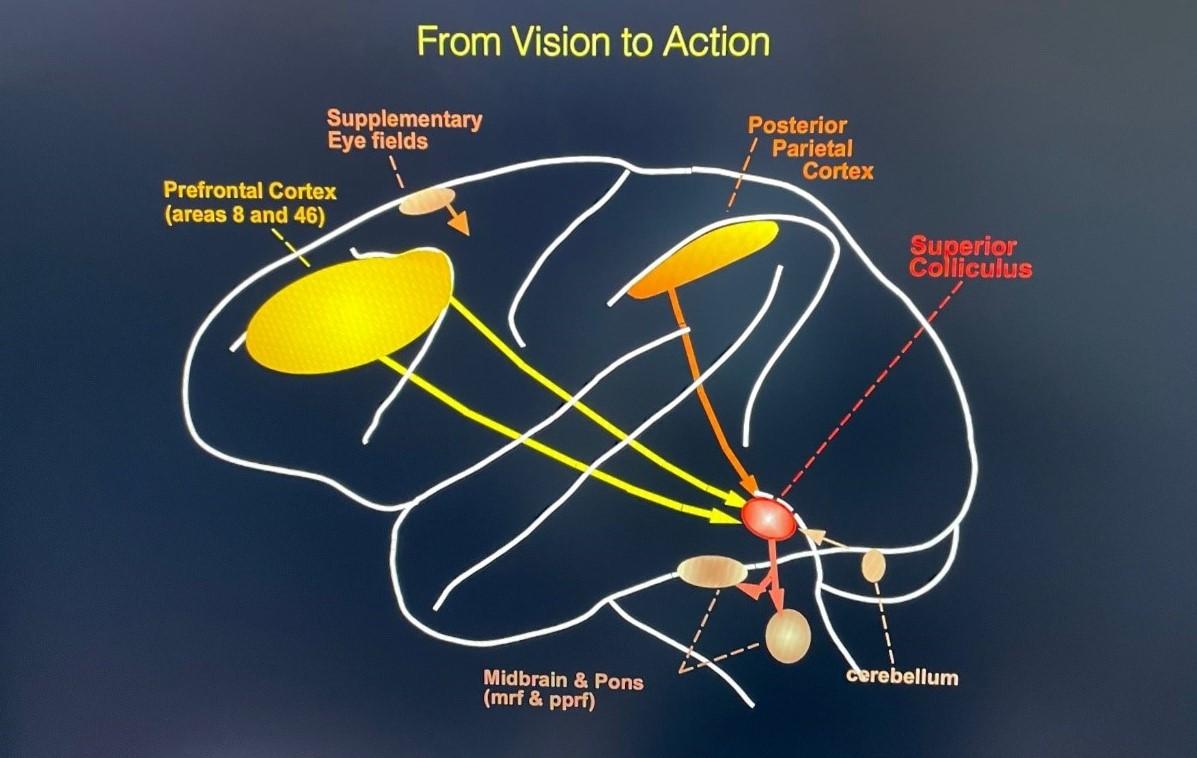
Why has it been challenging to understand the brain areas involved in how people combine past experience and sensory information?
It’s challenging on a number of levels. Firstly, it’s a very complicated process. It seems simple – we do it effortlessly without even thinking about it – but it requires a fair bit of computational power to do something like this. We have a lot of theoretical principles – statistical principles like Bayesian statistics and inference, for example – that help guide us. And we have a lot of behavioural (psychophysical) experiments that we can do with humans and other animals to see how they behave in certain situations to try to gain insight into what the computations might be. Then, we can rely on some of the Bayesian and other statistical advances to try to develop a more theoretical computational framework for how this might happen and make predictions. These things are well in place, but the next step is converting that theoretical, mathematical, computational ideas to a biological experiment. ‘How might the brain do this and how can I develop an experiment test this mechanism biologically?’ That part is tricky!
Based on many years of fundamental science, looking at the role of brain areas in different behaviours, we hypothesise that some brain areas are more or less likely to be involved based on what we know from other animal work and work with humans – stroke victims, for example. Then, once we identify those brain areas, we need to come up with the experiments to determine whether the brain areas are doing what we think they are doing.
What tools can we use to do this? Over the last 10-15 years or so we’ve made significant progress in tool development and that’s been in large part because of the funding that’s come through from the BRAIN Initiative that President Obama launched during his administration in the US. That initiative has generated many new creative tools that people have developed and built because it provided funding to allow scientists, engineers, and physicists to work together. Now we have a lot of tools to use to manipulate neuron types as well as to image and visualise neurons in action – and many of them at the same time, at very high individual-cell resolution. It’s amazing what we can see in the brain with these new tools. There are a whole host of experiments that can be done and questions that can be answered that we could have only dreamt about 10-15 years ago. That work is just getting going as we perfect the tools!
Now, what limits me is the development of these tools in monkeys. I believe very strongly that the mouse and rat are extraordinarily fantastic models for many scientific questions. However, when we’re starting to move into questions of higher mental function and psychiatric illness like disorders of perception, we need an animal model that likely has some of the same perceptual experiences as well as the sophistication in cognition that gets closer to a human. This would be the case if understanding humans and human brain disease is what we’re after. If we really want to understand the human experience, we need to study a species that more closely resembles a human in terms of cognitive sophistication. And in my strongly held opinion, that’s the monkey.
The limitation for me is getting these tools working so that I can do some of the same experiments in a monkey that people are doing in a mouse. While the experiments in mice have yielded very interesting results, it is not always clear how translatable they are to the human condition. There’s no stronger evidence of this than the Alzheimer’s disease story and, recently, COVID. The immune system is extremely different in mice than in primates. In order to study COVID in a mouse, you need to build a new mouse: a transgenic mouse that has a particular receptor. Mice don’t inherently have that receptor, unlike monkeys and humans. That’s why monkeys have played such a pivotal role in the rapid development of the vaccines. We can learn about the basic cell biology in transgenic mice, but it’s not the same. The same is true for the brain, perhaps even more so, because of the behavioural and cognitive sophistication.
How much is known about the changes to the brain that occur in people with Parkinson’s?
There’s a long history since the ‘50s and ‘60s when it was discovered that the neurotransmitter dopamine played a key role in the motor symptoms of Parkinson’s disease. Then in the ‘70s, there was a discovery of a compound, called MPTP (1-methyl-4-phenyl-1,2,3,6-tetrahydropyridine), by a group of people in San Francisco who were drug addicts and trying to synthesise their own drug. They made a mistake and created a compound called MPP (1-methyl-4-phenylpyridinium), and when injected, it converted into MPTP and within days destroyed all the catecholamine (dopamine) neurons within their brains. They had essentially acquired Stage 4 Parkinson’s disease. This discovery revolutionised the study of Parkinson’s disease – we now had a compound that we could use to create an animal model.
People are now working very hard on developing newer models, my laboratory included, to try to understand the degenerative process. As you imagine with this toxin, you inject it and the cells die very quickly. That’s not the disease, that’s the late-stage symptom. What we want to model is the disease, the slow progression of neuronal degeneration that happens even before visible symptoms arise. So there’s an aggressive effort to try and develop animal models of that degenerative process because if we can identify the disease process sooner, we might be able to develop treatments that would intervene sooner. This may prevent further degeneration, which would effectively be a cure. Slowing the progression gives people more days and a quality of life.
Can you please outline the novel, perceptual decision-making task you developed to study the influence of past experience on decision-making? What did this task help you discover about people with Parkinson’s?
The task was developed by a postdoctoral fellow in my lab at the time, Dr Alessandra Perugini. She used glass patterns, which are stimuli made up of a bunch of dots. We could vary the dots, that are linked together (correlated), and make the appearance of the stimulus very noisy, i.e. a bunch of dots scattered around the screen, or we could make it so that it looked very clearly like the pattern was tilted one way or the other. The participant then had to decide whether the dots were tilted to the left or to the right, and that decision was more or less difficult depending on the correlation of the pattern of the dots.
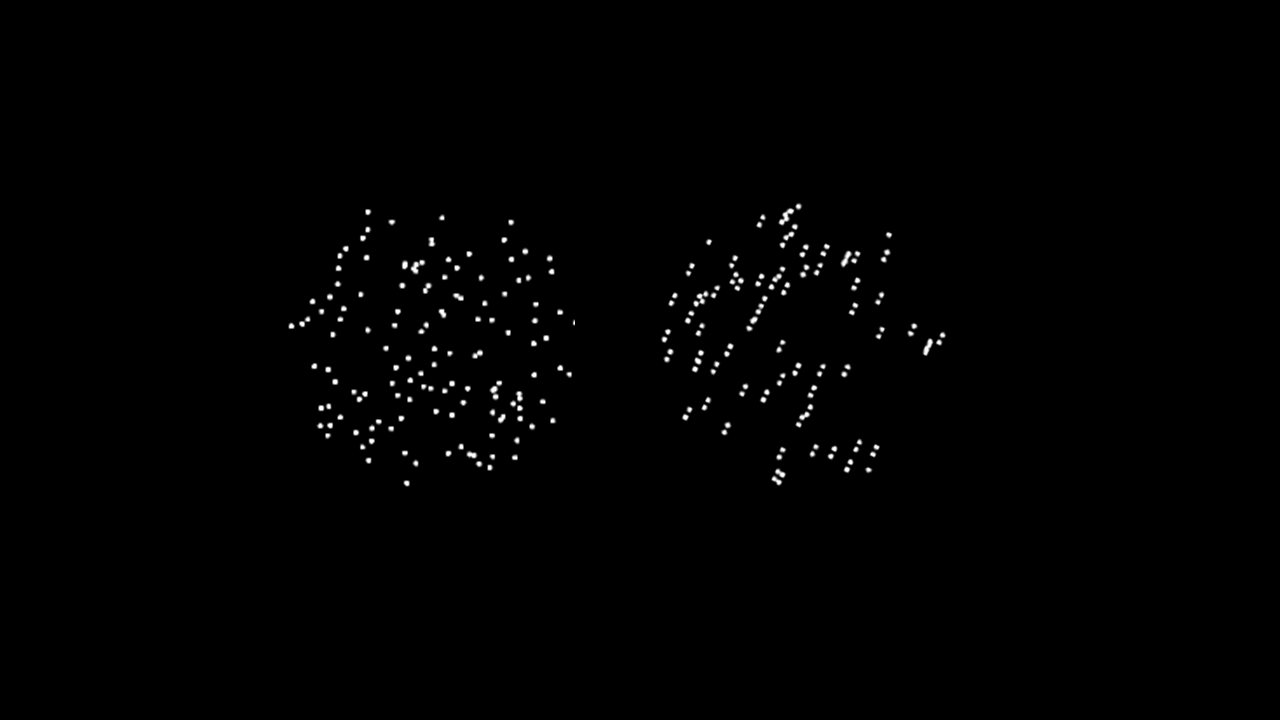
Next we provided a statistical rule that we didn’t tell the subjects about, but they learned implicitly: Alessandra presented these stimuli over hundreds of trials and in the overall group of trials, around 80% occurred with pattern tilt direction to the right, whereas 20% occurred with it tilted to the left. It took a couple hundred trials, but the healthy participants picked up on this – not necessarily consciously. In those trials where they weren’t quite sure what the tilt direction was, they relied on that statistic, or the memory of that 80:20 rule.
But people with Parkinson’s disease could not use that memory information. So this led us to think that the impairment that they have is related to this ability to integrate memory and sensory information and use that information when it’s needed. It’s similar to some of the motor impairments that people with Parkinson’s disease experience, called paradoxical movement. People with Parkinson’s have a stooped posture and a shuffling pattern to their gait, which we call festination. But if you put high contrast horizontal lines on the floor, many patients can actually generate a normal stride length: they can step over those lines. So it’s as if when the strong visual information is there, they can do it, but when the visual information is unclear (ambiguous) or absent, they can’t. This a fundamental impairment of motor ability in people with Parkinson’s.
We discovered what looks essentially like the same problem, but in the cognitive domain. When the information is based on clear sensory information, they can do it. But when they have to use that past experience to help them make that decision, they have trouble. So we’re beginning to think that this may be a fundamental problem in the symptoms of Parkinson’s disease. If we can understand the brain mechanisms behind that, we might be able to develop earlier, better diagnostic tools or potential treatments.
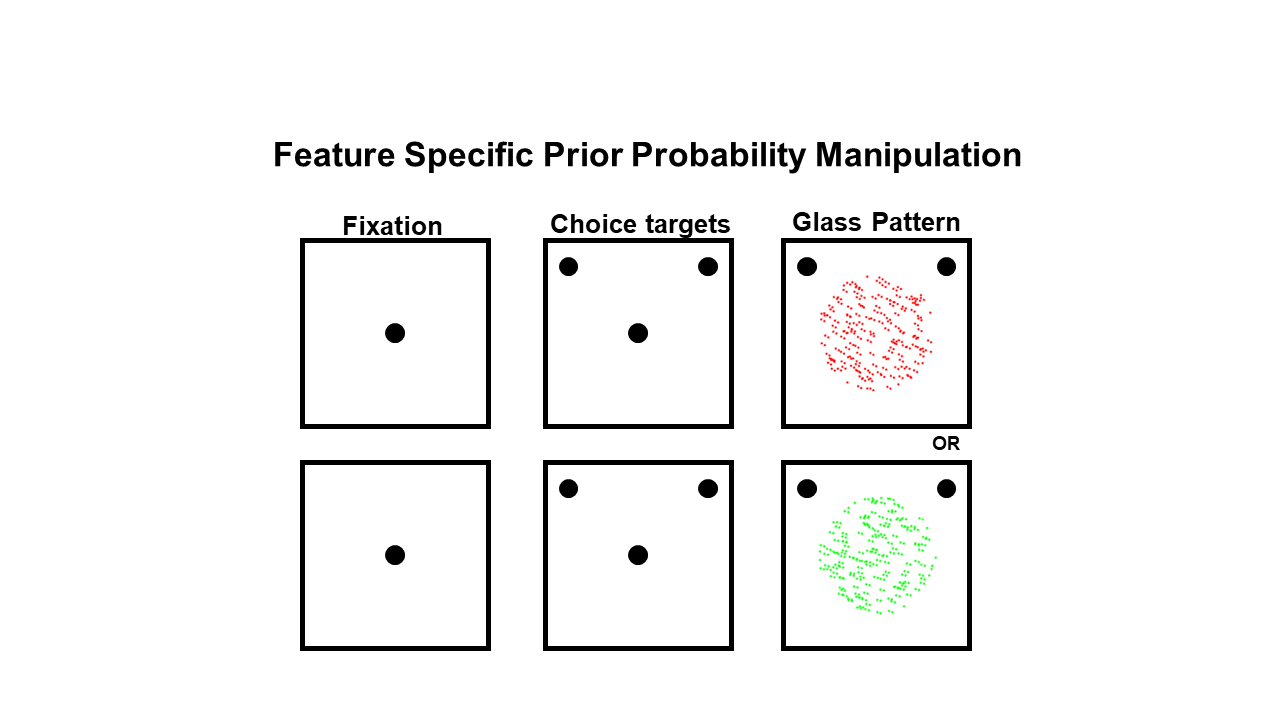
How did you determine that people with Parkinson’s couldn’t integrate memory and sensory information and not that they couldn’t learn the statistical rule?
For one experiment, Alessandra wanted to rule out that they couldn’t learn anything. One problem in Parkinson’s is that dopamine is known to be involved in reinforcement learning. So you might argue that the reason they couldn’t use these priors is because they couldn’t learn the reinforcement (reward) contingency. When Alessandra actually told them the statistical rule, much to our surprise, they still couldn’t use that information although healthy people could when they were told. And when they left the room, we asked them, ‘Did you remember what we told you when you first came in?’– and they knew! But they couldn’t use it, which was fascinating.
How did this lead you to explore how decision thresholds are established in the brain? Does this research have translational implications?
We engaged with our colleague Dr. Jochen Ditterich at UC Davis to do a modelling exercise. Jochen took the data from people and modelled it using a contemporary popular decision-making model called the drift diffusion model. This model has parameters that are thought to instantiate different processes related to decision-making. The parameters reflect: visual processing time, motor initiation time, the decision threshold (at what point you decide you’ve got enough information to make a decision), the slow accumulation of sensory evidence to reach a decision, and the integration of memory with sensory information. All those parameters are represented in this rather simplistic but very powerful model.
Jochen modelled the data for healthy people (age and sex-matched controls) and for people with Parkinson’s disease and he looked at what parameters of the model were different between the two. This led us to the prediction that the problem was in a decision threshold. In other words, the patients were impaired at knowing how much information to accumulate before making a decision.
This finding is consistent with some work done by others on people with Parkinson’s wherein they look at the influence of Deep Brain Stimulation. In these people with Parkinson’s disease, neurosurgeons treat the symptoms by implanting electrodes in the brain and when electrically stimulated, the electrodes miraculously treat some of the symptoms of Parkinson’s. People have looked at decision-making performance in these patients with and without their stimulation and they find that the stimulation actually affects how much information needs to be acquired for a decision so it’s consistent with the idea that part of the damage that comes with Parkinson’s disease is this inability to adjust decision thresholds. Again, this may be related to their motor impairment: they may have trouble initiating movement because they can’t adjust the decision for an action. So we actually think the cognitive impairments that we’re discovering are providing insight into the motor impairments that impact peoples’ lives.
What is the next piece of the puzzle your research is going to focus on?
Right now, we’re doing some basic physiology to understand the relationship of some brain areas to these decision-making processes. We’re doing electrophysiology in the basal ganglia and also in some of the places that this area communicates with, including a structure called the superior colliculus. We’re also trying to develop a new monkey model of Parkinson’s disease and rather than using MPTP, we’re using preformed alpha-synuclein fibrils, which is a protein element that is thought to be involved in the degenerative process in Parkinson’s disease. We hope to be able to mimic the disease process rather than the late stage symptom that current toxins mimic. This will allow longitudinal, long term, experiments to track the course of degeneration using non-invasive imaging and behavioural assays and ultimately electrophysiology as well.
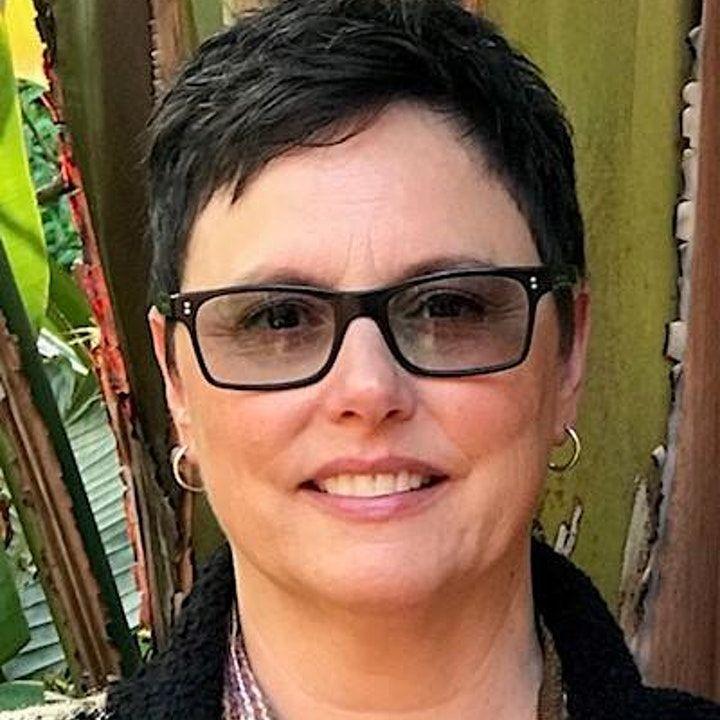
About Dr Michele A Basso
Dr Michele A. Basso is a Professor in the Departments of Psychiatry and Biobehavioral Sciences and Neurobiology. She studied Neuroscience at Stony Brook University in NY working with Dr. Craig Evinger and was a post-doctoral fellow at the National Eye Institute, NIH working with Dr. Robert Wurtz. After serving as a faculty member at the University of Wisconsin Madison, she moved to UCLA to join the faculty and direct the Fuster Laboratory of Cognitive Neuroscience. The work performed in her laboratory is aimed at unravelling the neuronal circuits of decision-making in health and disease. Her work spans multiple species and employs multiple technologies designed to understand how memory and sensory information are combined to give rise to our decisions.