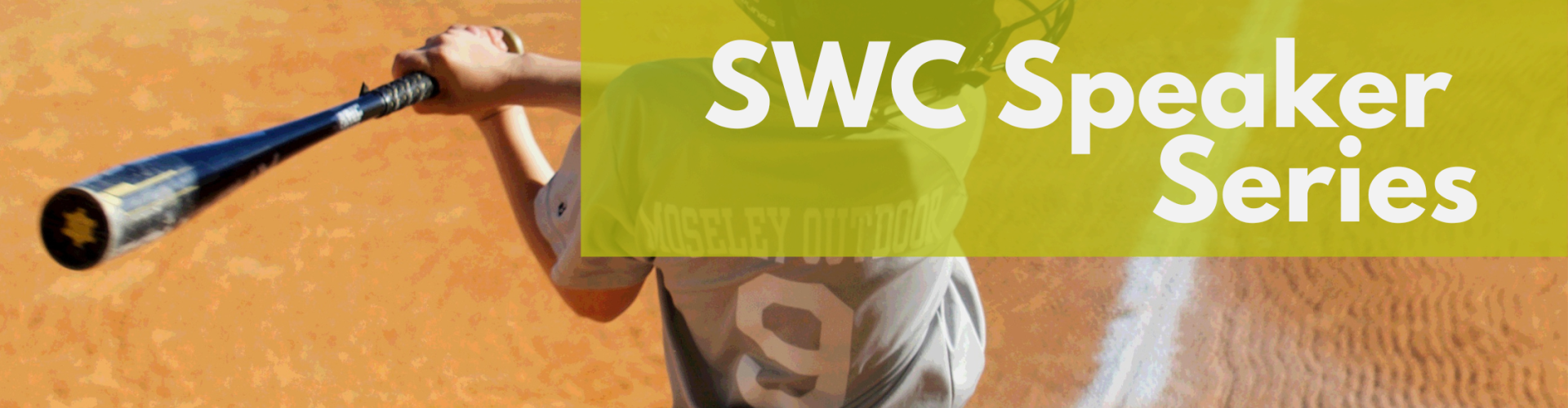
Preparing to make learned movements
An interview with Dr Takaki Komiyama, University of California San Diego, conducted by Hyewon Kim
Whether we’re articulating words to share with our family how the week went, getting on our bike to ride along the river, or envisioning a baseball player hit a home run, learned reproducible movements are an integral part of our lives.
In a recent SWC Seminar, Dr Komiyama shared his work showing how learned activity patterns in the brain’s primary motor cortex are causally related to the body generating a learned movement. In this Q&A, he elaborates on the role of a preparatory network activity state in the motor cortex, his dream experiment come true, why professional baseball players may play around with their batting gloves, and more.
Why were you initially interested in studying motor learning?
One of the fundamental functions of the brain is to move the body. This is highlighted in cases like Locked-In Syndrome, where most of other brain functions are normal, but one has lost the ability to move the body. Without this ability, you cannot communicate with the external world. Moving your body is the primary way to interact with and act on your surroundings. This includes speaking, gesturing, or making facial expressions.
Thus, moving the body is one of the most fundamental functions of the brain. And a lot of the movement we make arise from trial and error. This is called motor learning, or learning to move your body in an adaptive manner. My lab chose to study motor learning because of its fundamental nature as described above, and because my lab was a lab interested in studying the different aspects of learning in the first place.
What are some examples of learned movements in our daily life?
There are a lot of movements you make voluntarily rather than reflexively. And there is always some learned component. Sports are some good examples. Whether it’s tennis, golf, basketball, or riding a bike, you practice many times and your brain learns the ability to move your body in an adaptive way that reliably achieves your goal. This can be hitting the ball or putting the ball in the net. These are examples of learned motor skills.
How did you go about studying the mechanisms of motor learning in the motor cortex in your lab?
The motor cortex is known to be a central locus of larger brain circuits that are invoked in body movements, especially learned movements. And we use a number of optical technologies to study the functions of the motor cortex. Our approach is to use mice and allow them to learn some simple movements. In our case, we focus on forelimb or arm movements. In other words, mice learn to move their arm in a certain way to receive a reward. We combine that with optical techniques, such as high-resolution imaging to look at changes that happen in the brain’s motor cortex while the mice are learning a skill.
In your talk, you mentioned single cell resolution optogenetic stimulation as having been your dream experiment back in the day. Why was this your dream experiment?
Over the last decades, we have focused our efforts on trying to describe the changes that happen in the brain during learning. Using imaging, we observed these changes in the brain while the mice learned something. We have been trying to read the activity as well as structure of the brain.
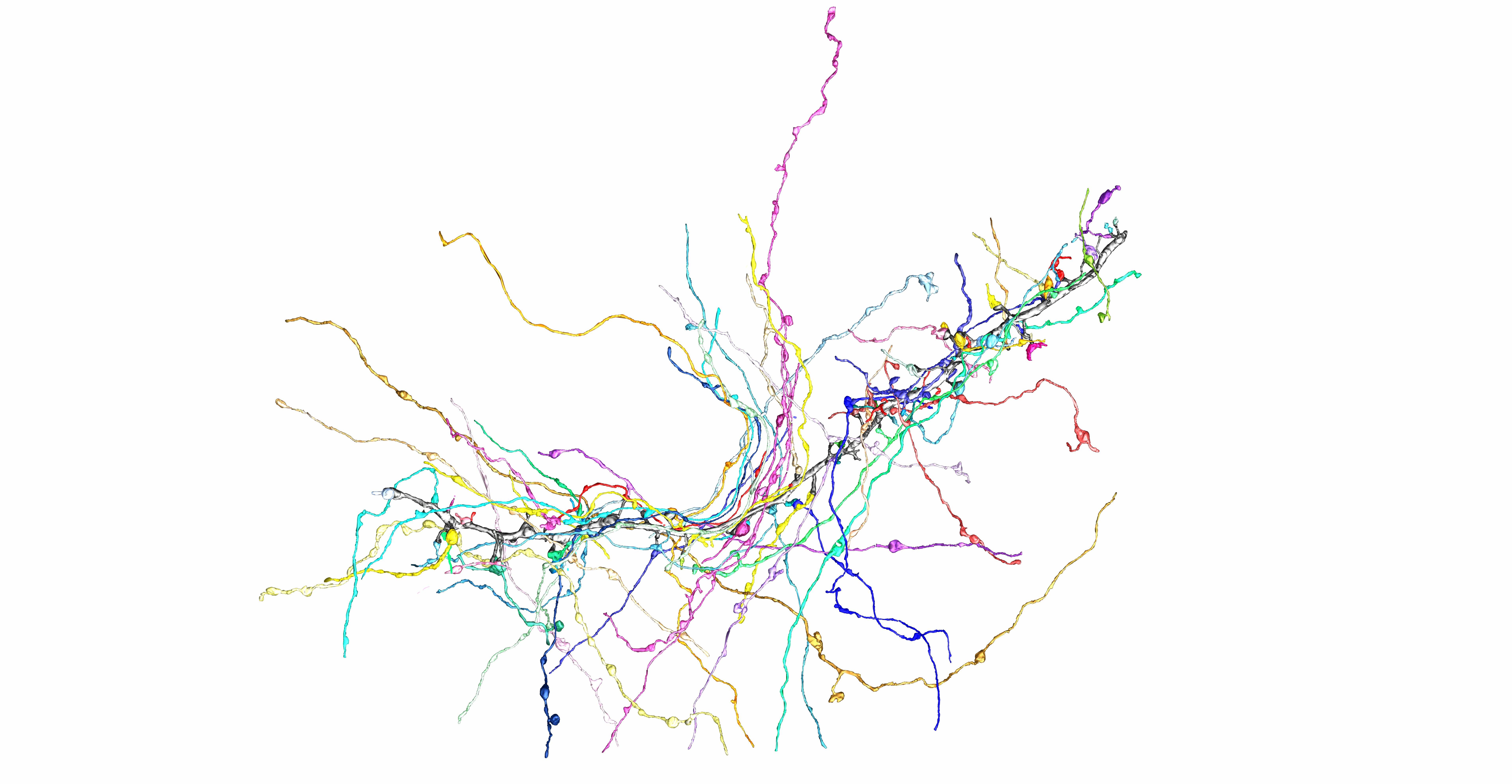
The limitation in this approach is that it is all correlational. We know these things happen during learning, but it creates a chicken-and-egg problem. Are these changes happening to cause the learning, or are these changes happening as a result of the learning? Or maybe it has nothing to do with learning – it just happens at the same time, but is related to other aspects of behaviour. These have been the uncertainties when describing our results.
Now, the new technologies that have become available in the last few years allow us to test the causality of the changes we’ve been observing. We have the ability to write specific activity patterns into the brain and see how that might be able to cause, or at least influence, the ongoing behaviour. That is why it’s an experiment we have been dreaming about for a long time! But the techniques have just become available in the last few years.
What did you find from these experiments?
What we have learned in the past 10 years is the following. In our paradigm, mice are able to learn to move their arm, or forelimb, in a reproducible way. An analogy would be how professional golfers swing their golf club in a reproducible way. And when the mice learn the reproducible movement, the brain and especially the primary motor cortex generates a reproducible activity pattern. In other words, the activity pattern is very similar from trial to trial.
We learned two things about how the brain generates a reliable activity pattern that drives the learned movement. First, the primary motor cortex has to prepare for the upcoming movement by entering a particular activity state. So certain neurons have to be slightly more active than baseline for the upcoming movement to successfully be the learned one. Second, the primary motor cortex has to receive a reliable input from another source.
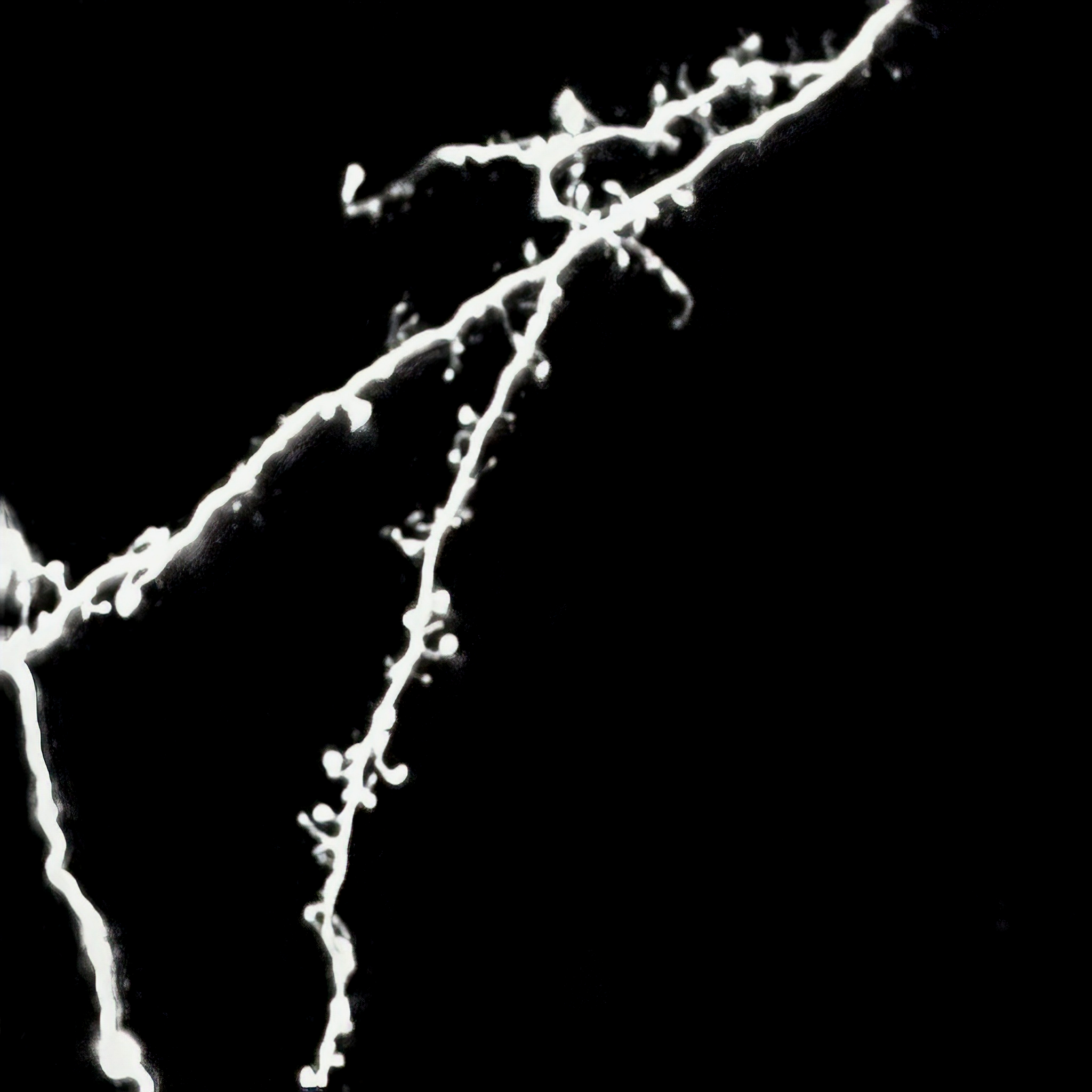
In our experiments, we are providing inputs to the motor cortex artificially by stimulating a small group of neurons. In a natural setting, another brain region would be activating those neurons. The primary motor cortex would have to receive an input to activate a certain group of neurons. So when it receives the input to activate a certain group of neurons and the cortex is prepared by having a certain preparatory activity pattern – when those two things happen at the same time – the primary motor cortex can generate the reliable learned activity pattern. That in turn drives the learned arm movement.
What could this preparatory network state mean in the context of learning? Was that at all surprising to you?
If you step back a little bit, it makes a lot of sense. Professional athletes are often known to have some routine movements before they make the key movements. These routine movements seem to be unrelated to the actual goal of the key movement. For example, baseball players would play around with their batting gloves or take fixed steps to enter the box – even taking the exact same steps every time. They have learned to do these things to prepare the brain to enter the reproducible state. This would involve the motor cortex, and perhaps other areas as well.
What will the next piece of the puzzle be for your team?
It is always the case that when we make a discovery, it leads to more questions than answers. There are many things we’d like to know. For instance, how widespread across the brain is this preparatory state? Is it something we see only in the primary motor cortex, or is it a brain-wide state from which we can decipher preparedness by looking at any part of the brain? Or maybe we can learn more about the preparedness by looking at interactions across brain areas.
What is the key mechanism behind the preparation – how does the brain enter the appropriate preparatory state? We have some guesses about what the mechanisms could be, but at the moment they are all speculations. And from a network simulation perspective, what is the mechanism by which appropriate preparation and inputs work together to interact with the local network, to generate the learned activity pattern? What is the structure of the local network that can translate these two things to the actual execution of the activity and body movement?
These are questions we hope to use data science and machine learning approaches to answer, and to figure out the precise nature of the network that can generate that learned behaviour.
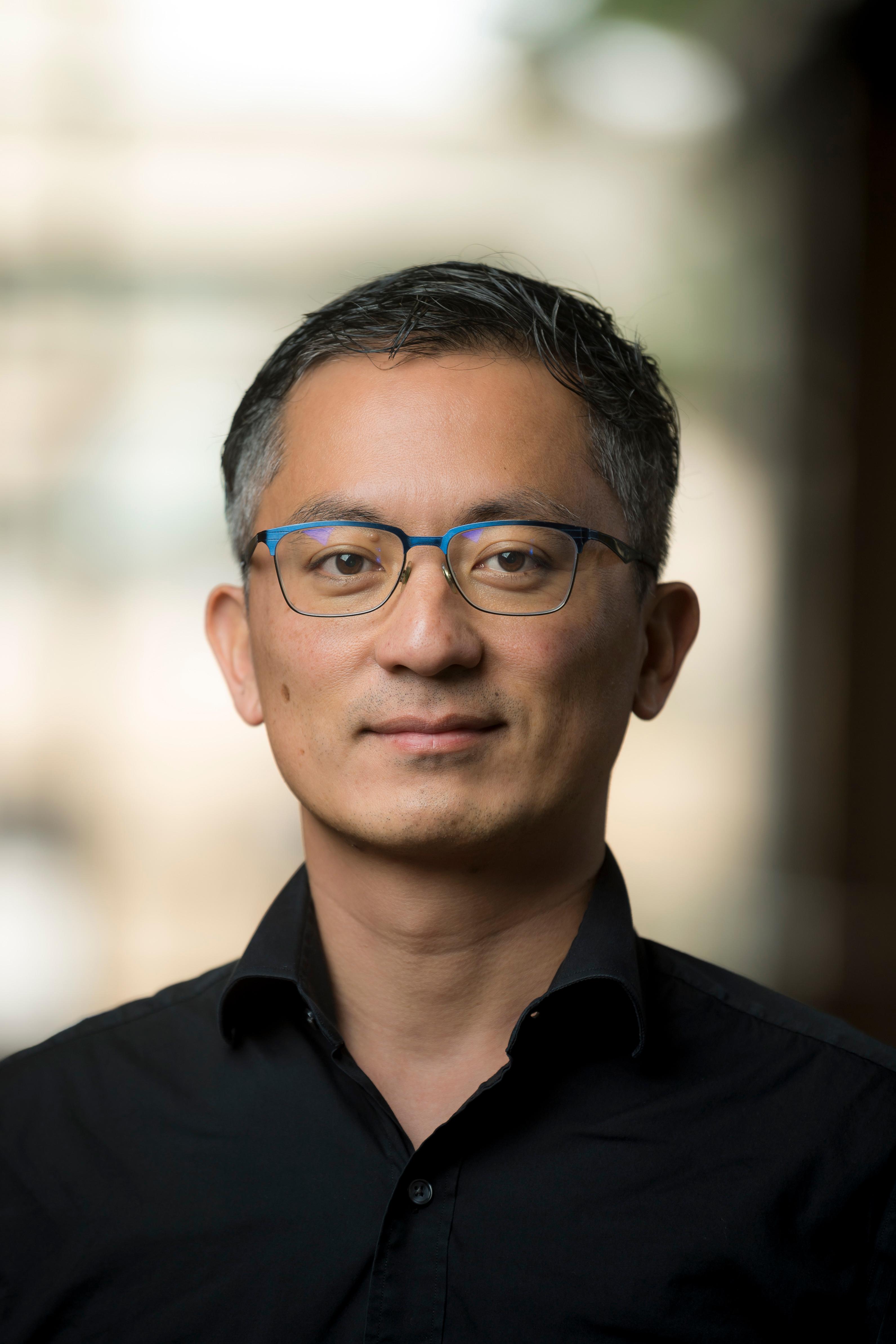
About Takaki Komiyama
Dr Komiyama was a Postdoc at Janelia Farm, Howard Hughes Medical Institute, received his PhD in Neurosciences at Stanford University (2006) and his BA in Biochemistry at University of Tokyo (2001). His Honors include: Helen Hay Whitney Foundation Postdoctoral Fellowship, Harold M. Weintraub Graduate Student Award, Japan-Stanford Association Graduate Fellowship, Stanford Graduate Fellowship, Pew Scholars Program, Packard Fellowship and Sloan Research Fellowship. Dr Komiyama is a NYSCF-Robertson Investigator.